Charge Your EV with Solar Power: Practical Complete Guide 2024
- Introduction: The Value and Viability of Charging Your EV with Solar Power
- Introduction: The Value and Viability of Charging Your EV with Solar Power
- Cost Savings That Matter
- Environmental Impact and Energy Independence
- Current Trends Driving Adoption
- What This Guide Will Do for You
- Technical Foundations and Prerequisites for Solar EV Charging
- Technical Foundations and Prerequisites for Solar EV Charging
- Core Hardware Components: Solar Panels, Inverters, and EV Chargers
- Electrical System Requirements and Configurations
- Regulatory and Energy Consumption Considerations
- Summary
- Calculating Your Solar Needs: Panel Sizing, Energy Usage, and System Design
- Calculating Your Solar Needs: Panel Sizing, Energy Usage, and System Design
- Estimating Your Daily Energy Consumption
- Considering Geographic and Environmental Factors
- Translating Energy Needs into Solar Panel Capacity
- Comparing Home-Only vs. Home + EV Solar Requirements
- Practical Performance Metrics and System Design Considerations
- Final Thoughts
- Installation and Integration: From Solar Panels to EV Charger Setup
- Installation and Integration: From Solar Panels to EV Charger Setup
- Solar Panel Installation: Roof vs. Carport Mounting
- Wiring Essentials and Electrical Connections
- Level 2 EV Charger Installation and Electrical Load Management
- Battery Storage Integration: Charging When the Sun Isn’t Shining
- Smart Charging Technologies and Home Energy Management Systems (HEMS)
- Final Thoughts
- Performance Insights and Real-World Usage: Comparing Technologies and Setups
- Performance Insights and Real-World Usage: Comparing Technologies and Setups
- Direct DC Charging vs. AC Inverter-Based Systems: Speed and Efficiency
- Energy Savings and Grid Interaction: Real-World Numbers
- Tackling Intermittency: Battery Storage and Bidirectional Charging
- Case Studies: Typical Usage and Cost Outcomes
- Final Thoughts
- Common Challenges and Troubleshooting Your Solar EV Charging System
- Common Challenges and Troubleshooting Your Solar EV Charging System
- Technical Challenges: Insufficient Solar Generation, Inverter Mismatch, and Charger Compatibility
- Practical Solutions: Maintenance, Correct Sizing, Software Updates, and Battery Care
- Monitoring System Health and Managing Energy
- Navigating Utility Restrictions and Maximizing Net Metering Benefits
- Advanced Optimization and Future-Proofing Your Solar EV Charging Setup
- Advanced Optimization and Future-Proofing Your Solar EV Charging Setup
- Smart Home Integration and AI-Driven Energy Management
- Oversizing Solar Systems for Future EVs and Household Loads
- Vehicle-to-Grid (V2G) Technology and Scalable Equipment
- Maximizing ROI and Environmental Impact Over Solar Panel Lifespan
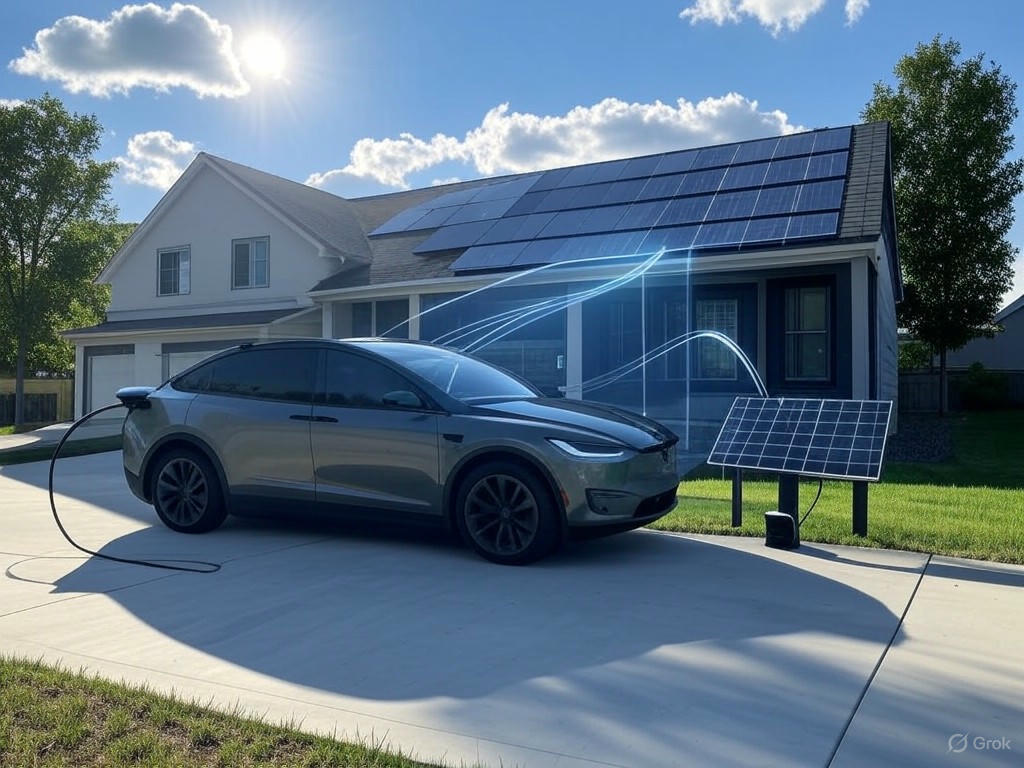
Introduction: The Value and Viability of Charging Your EV with Solar Power
Introduction: The Value and Viability of Charging Your EV with Solar Power
Charging electric vehicles (EVs) with solar power is no longer a futuristic idea—it’s a practical and rapidly growing reality in 2025. The combination of solar energy and EV technology offers substantial benefits beyond environmental ideals, including significant cost savings, enhanced energy independence, and a marked reduction in carbon emissions.
Cost Savings That Matter
Solar-powered EV charging can dramatically lower your energy expenses. For instance, SolarEdge’s latest solar-first EV charger technology can reduce fleet charging costs by up to 70% by prioritizing excess solar generation over grid electricity. On the residential side, solar panels typically help homeowners save approximately $125 per month on electricity bills, amounting to roughly $1,500 annually. Over the typical 25-year lifespan of solar panels, this translates to more than $16,000 saved on EV charging alone.
Unlike utility prices that fluctuate, solar energy locks in costs at around $0.06 per kWh in sunny areas like Florida—significantly below average grid rates. Modern solar panels last 25 to 30 years and retain about 80% of their efficiency over that period, insulating EV owners from rising energy costs. When compared to traditional gasoline or public charging stations—which can be 74% to 81% more expensive—solar EV charging becomes even more financially compelling.
Environmental Impact and Energy Independence
One common critique of EVs is that their electricity often derives from fossil fuels. Solar charging directly addresses this by dramatically reducing the carbon footprint of EV charging. Solar rooftop panels produce roughly 12 to 20 times less carbon than natural gas or coal power sources, enabling truly clean transportation fueled by renewable energy.
Beyond emissions, solar-powered EV charging enhances energy independence and resilience. Grid outages have increased by 80% over the past decade, highlighting the value of pairing solar panels with home batteries or bidirectional chargers. These systems can maintain power to your EV and home during outages, adding a crucial layer of reliability and security to your energy setup.
Current Trends Driving Adoption
Solar power is surging as a cornerstone of global clean energy. In 2024, clean energy sources accounted for over 40% of worldwide electricity generation, with solar leading new capacity additions for the third consecutive year. The U.S. solar market experienced a 20% increase in installations in Q2 2023 compared to the previous year, fueled by advances in panel efficiency and supportive policies.
EV sales are also accelerating, with nearly 300,000 new vehicles sold in the U.S. during Q1 2025. Major automakers continue to expand their EV offerings, broadening consumer choice and adoption. This simultaneous growth in solar and EV markets makes combining the two into integrated energy solutions more accessible and attractive than ever.
What This Guide Will Do for You
This guide cuts through the hype to provide a clear, practical roadmap for setting up your solar EV charging system. We will cover everything from calculating the number of solar panels you need to integrating home battery storage and Level 2 chargers. Our focus is on real-world conditions and solutions that fit both homeowners aiming to reduce their carbon footprint and energy bills, and fleet operators seeking operational savings.
By the end of this guide, you’ll understand what it takes to efficiently harness solar power for your EV. Drawing on the latest industry data and real user experiences, we’ll help you make informed, financially sound decisions that deliver lasting benefits. This is not just about protecting the planet—it’s about making smart investments that pay off for years to come.
Aspect | Details |
---|---|
Cost Savings | Up to 70% reduction in fleet charging costs (SolarEdge technology); ~$125/month or $1,500/year savings for homeowners; Over $16,000 saved in 25 years; Solar energy cost ~$0.06/kWh in sunny areas; 74%-81% cheaper than gasoline/public charging stations |
Solar Panel Lifespan | 25 to 30 years, retaining ~80% efficiency |
Environmental Impact | Solar rooftop panels produce 12 to 20 times less carbon than natural gas or coal; Enables clean transportation |
Energy Independence | Solar with home batteries/bidirectional chargers supports power during outages; Grid outages increased 80% over past decade |
Market Trends | Clean energy >40% of global electricity in 2024; Solar leads new capacity additions for 3rd year; US solar installations up 20% in Q2 2023; Nearly 300,000 new EVs sold in US Q1 2025 |
Guide Focus | Calculating solar panels, integrating home battery storage, Level 2 chargers; Practical solutions for homeowners and fleet operators |
Technical Foundations and Prerequisites for Solar EV Charging
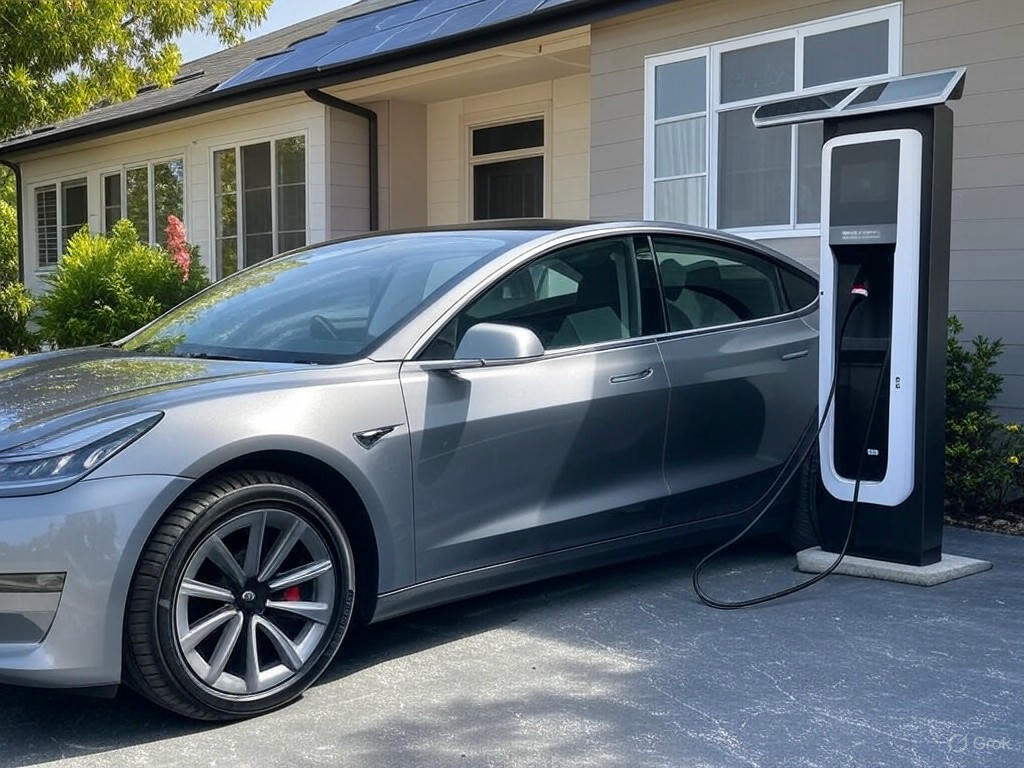
Technical Foundations and Prerequisites for Solar EV Charging
Charging your electric vehicle (EV) with solar power is more than an environmental aspiration—it’s a practical, technically sound solution that offers substantial cost savings and energy independence. To set up an effective solar EV charging system, it’s essential to understand the core hardware components, electrical requirements, system configurations, and regulatory incentives that underpin this technology.
Core Hardware Components: Solar Panels, Inverters, and EV Chargers
Solar Panels: Types and Efficiency
At the center of any solar EV charging setup are the solar panels themselves. As of 2025, the most advanced residential solar panels deliver between 370W and 465W per panel, with efficiencies surpassing 24% under Standard Test Conditions (STC). Leading technologies include:
- Back-contact (IBC) cells with N-type silicon substrates, reaching up to 24.1% efficiency. These designs reduce shading losses and enhance panel longevity.
- N-Type TOPCon and Heterojunction (HJT) cells, which offer efficiencies exceeding 23%, improved temperature coefficients, and slower degradation rates.
For typical home installations, monocrystalline panels remain the preferred choice due to their balance of high efficiency (generally 17–22%) and durability. In contrast, polycrystalline and thin-film panels, often below 18% efficiency, require larger arrays and more roof space, making them less ideal for residential solar EV charging.
Real-world conditions such as shading, panel orientation, and temperature can reduce output by 8–15% compared to laboratory ratings. For example, a nominal 400W panel may produce closer to 340–370W during sunny days.
Inverters: DC to AC Conversion
Solar panels generate direct current (DC) electricity, but your EV charger and household appliances operate on alternating current (AC). The inverter is responsible for converting DC to usable AC power. The right inverter improves overall system efficiency and compatibility.
- String inverters are widely used for their cost-effectiveness but can experience performance drops if panels face shading or have varied orientations.
- Microinverters and DC optimizers (e.g., SolarEdge) optimize output on a per-panel basis, ideal for complex roof layouts or partial shading.
- Reputable inverter brands include SolarEdge, Enphase, SMA, and Fronius, offering warranties of 10–12 years.
- Hybrid inverters facilitate seamless integration with battery storage solutions like the Tesla Powerwall 3.
Selecting an inverter depends on your solar array size, roof conditions, and plans for future energy storage expansion.
Level 2 EV Chargers: Efficient Home Charging
For residential solar EV charging, Level 2 chargers operating at 240 volts provide the optimal balance between charging speed and infrastructure cost.
- Typical Level 2 chargers deliver between 7.2 kW and 11.5 kW, with amperage ratings from 30A to 48A.
- Examples include the Emporia Pro EV Charger (48A) and Wallbox Pulsar Plus (up to 48A), both offering smart solar integration to prioritize solar energy consumption.
- Installing these chargers usually requires a dedicated 60-amp circuit to ensure safe operation.
- Level 2 chargers can fully charge most EV batteries within 4 to 8 hours, aligning well with daily solar production cycles.
In contrast, Level 1 chargers (120V) produce only about 1.2–1.4 kW, which is too slow for daily solar-powered charging needs.
Electrical System Requirements and Configurations
Voltage and Amperage Specifications
- Residential EV chargers generally require a 240V circuit.
- Amperage depends on the charger’s rating; Level 2 chargers commonly draw 30 to 48 amps.
- Electrical panels and wiring must be assessed and possibly upgraded to safely handle these loads, often involving installation of dedicated breakers.
Grid-Tied vs. Off-Grid Systems
Most home solar EV charging systems are grid-tied, allowing solar panels to feed excess electricity back to the grid and draw from it when solar production is insufficient.
- Grid-tied systems benefit from net metering policies, which credit exported solar power against your consumption, reducing utility bills.
- Off-grid systems operate independently of the utility grid and require energy storage solutions like the Tesla Powerwall 3 to store solar energy for nighttime or cloudy-day charging.
- Off-grid setups are more complex and typically incur higher installation costs.
Energy Storage Integration
Adding battery storage enhances energy independence and solar self-consumption:
-
The Tesla Powerwall 3 is a leading residential battery, featuring:
- 13.5 kWh usable capacity with lithium ferro phosphate (LFP) chemistry for improved safety and longevity.
- Continuous power output of 11.5 kW, sufficient for most household and EV charging loads.
- Round-trip efficiency around 89%, and solar-to-home/grid efficiency of 97.5%.
- Backup power lasting 10–12 hours for typical essential home loads.
- Advanced integration with solar and smart home energy management systems.
-
Batteries increase upfront costs and installation complexity but enable exclusive solar EV charging and resilience during outages.
Regulatory and Energy Consumption Considerations
Typical EV Energy Use and Solar Capacity Requirements
The average U.S. electric vehicle consumes approximately 4,666 kWh per year. To reliably cover this with solar energy, expect to install a minimum of about 3.1 kW of solar capacity.
- This capacity generally equates to 7 to 9 high-efficiency solar panels rated between 370W and 465W, accounting for real-world losses.
- Actual system size should be tailored to your driving habits, vehicle efficiency, local climate, and roof characteristics.
For perspective, a 2025 Porsche Macan EV achieves around 3.5 miles per kWh with a 95 kWh battery, requiring 9 to 12 kWh of daily charging energy depending on use.
Federal and State Incentives
Financial incentives significantly reduce the initial investment:
- The Federal Solar Tax Credit (Investment Tax Credit, ITC) offers a 30% tax credit on qualified solar installation costs through 2032, including solar panels and eligible EV charging equipment.
- Many states and utilities provide additional rebates or incentives that can be stacked with federal credits.
- These programs apply to both homeowners and businesses and often require specific documentation to claim.
Summary
To build a reliable solar EV charging system, prioritize:
- High-efficiency monocrystalline solar panels with wattages between 370W and 465W.
- A compatible inverter—string, microinverter, or hybrid type—aligned with your roof layout and storage plans.
- A Level 2 EV charger rated for 30–48 amps on a dedicated 240V circuit.
- Consider integrating battery storage solutions such as the Tesla Powerwall 3 for enhanced energy independence and off-grid capability.
Accounting for an average EV consumption of around 4,666 kWh annually, a solar array of at least 3.1 kW is essential, with oversizing of 10–20% recommended to accommodate future load growth and system losses.
Don’t overlook available federal and state incentives that can significantly offset costs. For optimal results, engage professional installers and ensure your system design suits your home’s unique characteristics, driving patterns, and local regulations.
With thoughtful planning and quality components, solar EV charging delivers both environmental impact and financial benefits that grow over decades of use.
Component | Details |
---|---|
Solar Panels |
|
Inverters |
|
EV Chargers |
|
Electrical Requirements |
|
System Types |
|
Battery Storage (Tesla Powerwall 3) |
|
EV Energy Use & Solar Capacity |
|
Incentives |
|
Calculating Your Solar Needs: Panel Sizing, Energy Usage, and System Design
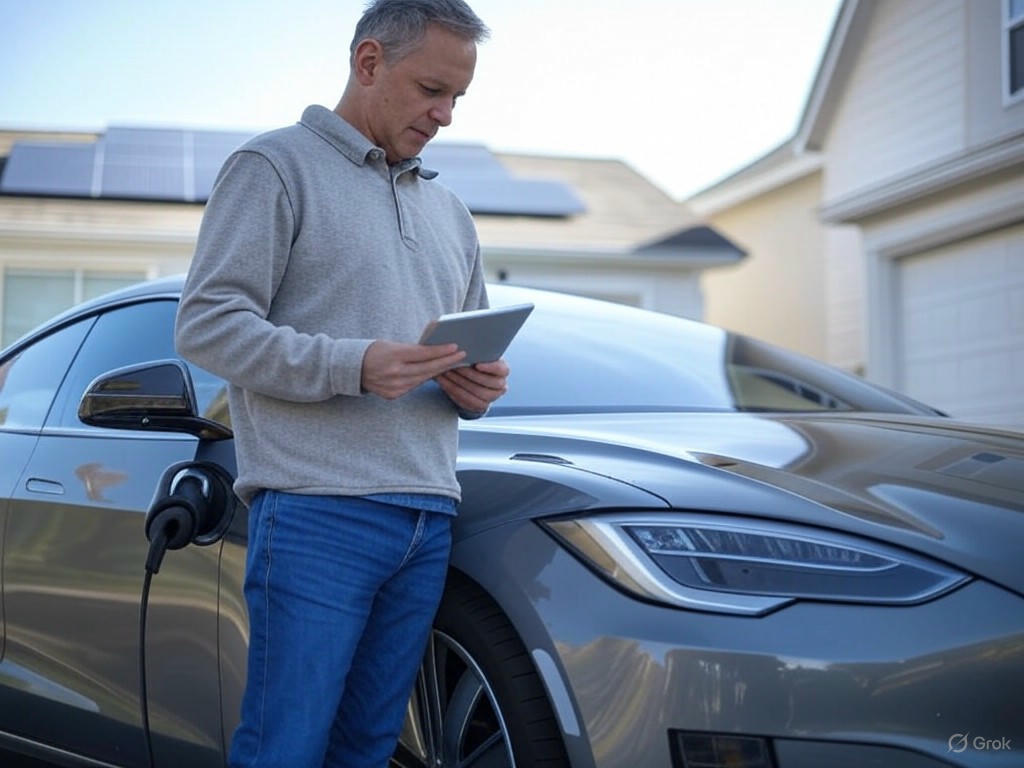
Calculating Your Solar Needs: Panel Sizing, Energy Usage, and System Design
Before investing in a solar setup to power both your EV and home, it’s essential to have a realistic understanding of your total energy needs and how these translate into solar panel requirements. This analysis goes beyond marketing claims—it requires a thorough look at your daily electricity consumption, EV charging patterns, and the environmental factors affecting solar output at your location.
Estimating Your Daily Energy Consumption
Begin by assessing two primary components: household electricity use and EV charging demand.
-
Household Energy Use: The average U.S. home consumes about 30 kWh per day, or roughly 900 kWh per month. This varies based on region, home size, and lifestyle. For example, households in states like Louisiana and Florida often use over 13,000 kWh annually due to higher cooling loads, while smaller or energy-conscious homes might consume less than 10,000 kWh per year.
-
EV Energy Consumption: EV energy use depends on the vehicle model and driving habits. For instance, the 2025 Porsche Macan EV averages approximately 3.5 miles per kWh, with a 95 kWh battery providing around 330 miles of range. If you drive 30 to 40 miles daily, expect to use about 9 to 12 kWh per day for charging.
Combined, a typical household with an EV may require between 40 and 50 kWh daily—significantly higher than a home without EV charging. This increase must be accounted for when sizing your solar system.
Considering Geographic and Environmental Factors
Your geographic location strongly influences the solar energy your system can generate. Peak sun hours vary widely across the U.S., ranging from about 2.5 hours in the Pacific Northwest to over 7 hours in the Southwest.
-
Peak Sun Hours: This metric represents the average daily sunlight intensity sufficient to produce 1,000 watts per square meter. Most U.S. areas receive between 3 and 5 peak sun hours daily. For example, California enjoys 5.5 to 7 peak sun hours, while New York averages 3 to 4.
-
Panel Orientation and Shading: To maximize solar exposure, panels should generally face true south with a tilt angle close to your latitude. Even minor shading from trees or nearby structures can reduce output by 5% to 25%, impacting annual energy yield. Technologies such as bypass diodes and bifacial panels can help mitigate shading losses.
-
Seasonal Variability: Solar production fluctuates seasonally; winter months may produce 30% to 50% less energy than summer. If you aim for year-round EV charging solely from solar, consider oversizing your system or integrating battery storage to compensate for these seasonal dips.
Translating Energy Needs into Solar Panel Capacity
To estimate the number of solar panels required for your system, follow these steps:
-
Calculate total daily kWh demand: Add your household and EV energy use (e.g., 45 kWh/day).
-
Determine your location’s average peak sun hours: For example, 5 hours in a sunny region.
-
Estimate system size in kW: Divide daily kWh demand by peak sun hours.
Example: 45 kWh ÷ 5 hours = 9 kW system. -
Calculate the number of panels: Divide the system size (in watts) by the wattage of each panel. Modern panels typically range from 370 W to 465 W.
Example: 9,000 W ÷ 400 W/panel ≈ 23 panels.
This aligns with typical data showing that average households require 15 to 20 panels for home use alone, while adding EV charging increases this number substantially.
Comparing Home-Only vs. Home + EV Solar Requirements
Adding EV charging to a home solar system typically increases the required solar capacity by 30% to 50%, depending on daily driving distance.
-
A 5 kW system (about 15 panels) generally covers a typical home’s 30 kWh/day electricity use.
-
Introducing an EV that consumes 10 to 15 kWh/day raises total demand to 40 to 45 kWh/day, necessitating an 8 to 11 kW solar system, or roughly 25 to 30 panels.
Keep in mind this is a simplified calculation. Real-world factors such as inverter efficiency (usually between 90% and 98%), panel degradation over time, and occasional cloudy days reduce actual output. Including a 10% to 20% oversizing buffer is a prudent design strategy to ensure reliable performance.
Practical Performance Metrics and System Design Considerations
-
Annual kWh Generated per kW Installed: On average, a 1 kW solar system produces between 1,200 and 1,500 kWh per year in the U.S., equating to about 3.3 to 4.1 kWh per day per kW installed, depending on location.
-
Panel Efficiency: Modern monocrystalline panels exceed 20% efficiency, with some bifacial models increasing output by up to 10% by capturing reflected light. Higher efficiency panels reduce the number of panels needed and save valuable roof space.
-
System Layout: Roof size, shape, and structural capacity limit how many panels you can install. If roof space is constrained, ground-mounted arrays or solar carports may be viable alternatives.
-
Electrical Panel Capacity: EV charging often increases your home’s electrical load. A professional electrician should assess your electrical panel’s capacity to handle this increased demand and recommend upgrades or smart load management solutions if necessary.
Final Thoughts
Charging your EV with solar power is both practical and environmentally responsible, but it requires careful upfront planning. Underestimating your energy needs or neglecting geographic and shading factors can lead to underperformance and continued reliance on grid electricity.
For most households with an EV, expect to install a solar array roughly 50% larger than a home-only system—typically 20 to 30 panels, depending on your daily driving habits and local sunlight availability.
By basing your system design on solid consumption data and realistic solar production estimates, you’ll avoid costly surprises and maximize your investment—delivering clean, reliable energy for both your home and your electric vehicle.
Category | Details | Values / Examples |
---|---|---|
Household Energy Use | Average daily and annual consumption | ~30 kWh/day, ~900 kWh/month, 10,000 to 13,000+ kWh/year depending on region |
EV Energy Consumption | Example: 2025 Porsche Macan EV; daily driving consumption | 3.5 miles/kWh, 95 kWh battery (~330 miles range), 9 to 12 kWh/day for 30-40 miles driven |
Total Daily Energy Need (House + EV) | Combined household and EV charging demand | 40 to 50 kWh/day |
Peak Sun Hours (U.S. Range) | Average daily sunlight intensity | 2.5 (Pacific NW) to 7 (Southwest), typical 3 to 5 hours |
Panel Orientation & Shading Loss | Optimal panel facing and shading impact | Panels face true south, tilt ≈ latitude; shading reduces output by 5%–25% |
Seasonal Variability | Winter vs. summer production difference | Winter produces 30% to 50% less energy than summer |
System Sizing Calculation | Example calculation for 45 kWh/day demand and 5 peak sun hours | System size = 45 kWh ÷ 5 h = 9 kW; Number of panels = 9000 W ÷ 400 W ≈ 23 panels |
Home-Only Solar System | Typical system size and panel count for home energy only | 5 kW system, ~15 panels, covers ~30 kWh/day |
Home + EV Solar System | Increased system size and panels with EV charging | 8 to 11 kW system, ~25 to 30 panels, covers 40 to 45 kWh/day |
Oversizing Buffer | Recommended system oversizing to offset losses | 10% to 20% additional capacity |
Annual Energy Production per kW Installed | Typical U.S. range | 1,200 to 1,500 kWh/year (3.3 to 4.1 kWh/day per kW) |
Panel Efficiency | Typical efficiencies and advanced panel types | Monocrystalline >20%, bifacial up to 10% higher output |
System Layout Considerations | Roof space and alternatives | Roof size/shape limits panels; ground mounts or solar carports possible |
Electrical Panel Capacity | Impact of EV charging on home electrical system | May require upgrades or smart load management by electrician |
Installation and Integration: From Solar Panels to EV Charger Setup
Installation and Integration: From Solar Panels to EV Charger Setup
Installing a solar-powered EV charging system is a technical but rewarding process that demands careful planning, strict adherence to electrical codes, and smart integration decisions. This section breaks down the essential steps and considerations, reflecting the latest 2025 standards and insights from experienced installers.
Solar Panel Installation: Roof vs. Carport Mounting
Choosing the right mounting option for your solar panels is the foundational step. The two primary residential options are rooftop and solar carport installations, each offering distinct benefits.
-
Roof Mounts are the most common choice among U.S. homeowners, who typically prefer south-facing panels to maximize solar energy yield. Installation generally takes between 1 and 3 days, depending on system size and roof complexity. Compliance with the National Electrical Code (NEC) is mandatory, including safety features such as rapid shutdown switches located near the panels for emergency access.
-
Solar Carports provide an excellent alternative when rooftop mounting is impractical due to shading, orientation, or structural limitations. These overhead canopies simultaneously shield your vehicle and generate solar power. Costs average around $3.17 per watt, translating to roughly $15,000 before tax credits for a typical 5 kW system. Although more expensive than rooftop systems, carports offer added vehicle protection and easier panel maintenance. Permitting is often required, and installation must consider the surface type—whether concrete, asphalt, or grass.
From practical experience, solar carports are ideal for homeowners seeking dual benefits: clean energy generation plus covered parking. Meanwhile, roof mounts remain the favored approach for maximizing solar density and minimizing upfront costs.
Wiring Essentials and Electrical Connections
Proper wiring is critical to an efficient and safe solar-to-EV charging setup. Key points include:
-
Using cables and connectors rated for the application and compliant with NEC standards to ensure safety and reduce energy losses.
-
Wiring configurations vary: series wiring increases voltage but is sensitive to shading, whereas parallel wiring enhances resilience to partial shading but requires thicker wiring.
-
String inverters are widely used to convert DC power from solar panels to AC for home use and EV charging. When integrating battery storage, the inverter also connects to the battery bank to manage energy flow.
-
Electrical load management is vital. Level 2 EV chargers, which operate at 240 volts, necessitate a dedicated circuit breaker—commonly rated between 40 and 60 amps depending on the charger’s specifications—to maintain safety and meet local code requirements.
Because of the complexity and safety implications, engaging a licensed electrician for wiring and connections is strongly recommended. Incorrect wiring or inverter misconfigurations can severely reduce system efficiency or create hazards.
Level 2 EV Charger Installation and Electrical Load Management
Level 2 chargers offer a practical balance between charging speed and home electrical capacity. Important considerations include:
-
Installation requires a dedicated 240V circuit with appropriate amperage, typically 40 amps or higher, enabling charging power from 7 kW up to approximately 9.6 kW.
-
Popular chargers such as the Tesla Universal Wall Connector and Emporia Level 2 models integrate seamlessly with home solar systems and include smart load management to prioritize solar energy use.
-
Many smart chargers feature mobile apps that allow scheduling charging during peak solar production or off-peak utility rate periods, alongside real-time energy monitoring.
-
Compliance with local electrical codes and permitting through the Authority Having Jurisdiction (AHJ) is mandatory. This includes adherence to NEC guidelines on breaker sizing, conduit installation, and grounding.
Testing shows that Level 2 chargers with integrated solar prioritization and load management—like the Enphase IQ 50 or Wallbox Pulsar Plus—offer superior cost savings and increased grid independence compared to basic units.
Battery Storage Integration: Charging When the Sun Isn’t Shining
Solar panels only generate electricity when the sun is shining. To enable EV charging overnight or during cloudy days, incorporating battery storage is essential:
-
Battery systems such as Tesla Powerwall 3 and Bluetti EP900 store surplus solar energy produced during the day, allowing EV charging at night without drawing from the grid.
-
Proper battery capacity sizing should reflect combined household and EV consumption patterns. For example, a 13.5 kWh Tesla Powerwall can cover a full night’s energy needs or power both home and EV loads during low-sun periods.
-
Lithium-ion batteries, especially lithium iron phosphate (LFP) chemistries, dominate residential solar storage due to high efficiency, long lifespans (typically 10–15 years), and strong safety profiles.
-
Integration requires compatible inverters and advanced energy management systems that seamlessly switch between solar, battery, and grid power to optimize performance.
Hands-on experience confirms that pairing a robust battery storage system with your solar array unlocks true energy independence and resilience during grid outages, although this entails a significant upfront investment—often exceeding $10,000 fully installed.
Smart Charging Technologies and Home Energy Management Systems (HEMS)
Modern solar-to-EV charging setups gain considerable benefits from smart technologies that optimize energy flow and cost savings:
-
Smart EV chargers connect via Wi-Fi or cellular networks and leverage AI with real-time data to schedule charging during peak solar output or low grid demand periods.
-
Home Energy Management Systems (HEMS), such as EcoFlow’s AI-driven platform or ELECQ’s smart load balancing solution, provide dynamic control of energy usage, balancing solar generation, battery storage, EV charging, and grid interaction.
-
These systems can reduce EV charging costs by 70% or more by prioritizing solar self-consumption, as demonstrated by SolarEdge’s EV chargers designed for solar-powered charging.
-
Integration with voice assistants like Alexa and Google Home, along with mobile apps, enables remote monitoring, scheduling, and participation in demand response programs.
Testing of smart EV chargers and HEMS shows that automating charging based on solar availability and utility rates delivers substantial real-world savings and enhanced user convenience beyond static charging setups.
Final Thoughts
Successfully setting up a solar-powered EV charging system involves multiple coordinated components: selecting the most suitable solar panel mounting method, ensuring safe and code-compliant wiring, choosing a Level 2 EV charger with smart solar integration, incorporating battery storage for off-sun charging, and leveraging advanced home energy management systems to optimize energy flow.
While the initial complexity and costs can be significant, the long-term financial savings combined with environmental benefits make this approach highly worthwhile. For most homeowners, professional installation paired with scalable, smart technology offers the best path to reliable, cost-effective solar EV charging.
If you aim to reduce your carbon footprint and secure low, predictable energy costs for years to come, this integrated installation and integration strategy is the practical and forward-looking solution in 2025 and beyond.
Installation Aspect | Details |
---|---|
Solar Panel Installation | Roof Mounts: South-facing preferred, 1-3 days installation, NEC compliance with rapid shutdown switches. Solar Carports: $3.17/watt (~$15,000 for 5kW), vehicle protection, easier maintenance, requires permitting, surface dependent (concrete, asphalt, grass). |
Wiring Essentials | Use NEC-compliant cables and connectors; series wiring (higher voltage, shading sensitive) vs. parallel wiring (shade resilient, thicker cables); string inverters for DC-AC conversion and battery integration; dedicated circuit breaker (40-60 amps) for safety; licensed electrician recommended. |
Level 2 EV Charger Installation | Dedicated 240V circuit, typically 40+ amps; charging power 7-9.6 kW; popular models include Tesla Universal Wall Connector, Emporia Level 2; smart load management and mobile apps; NEC and AHJ code compliance mandatory. |
Battery Storage Integration | Examples: Tesla Powerwall 3 (13.5 kWh), Bluetti EP900; stores surplus solar energy for night/cloudy day charging; lithium-ion (LFP) preferred for efficiency, lifespan (10-15 years), safety; requires compatible inverters and energy management; upfront cost > $10,000 installed. |
Smart Charging & HEMS | Wi-Fi/cellular connected chargers with AI scheduling; HEMS like EcoFlow, ELECQ for dynamic load balancing; reduce charging costs by 70%+ via solar prioritization; voice assistant integration; remote monitoring and demand response participation. |
Performance Insights and Real-World Usage: Comparing Technologies and Setups
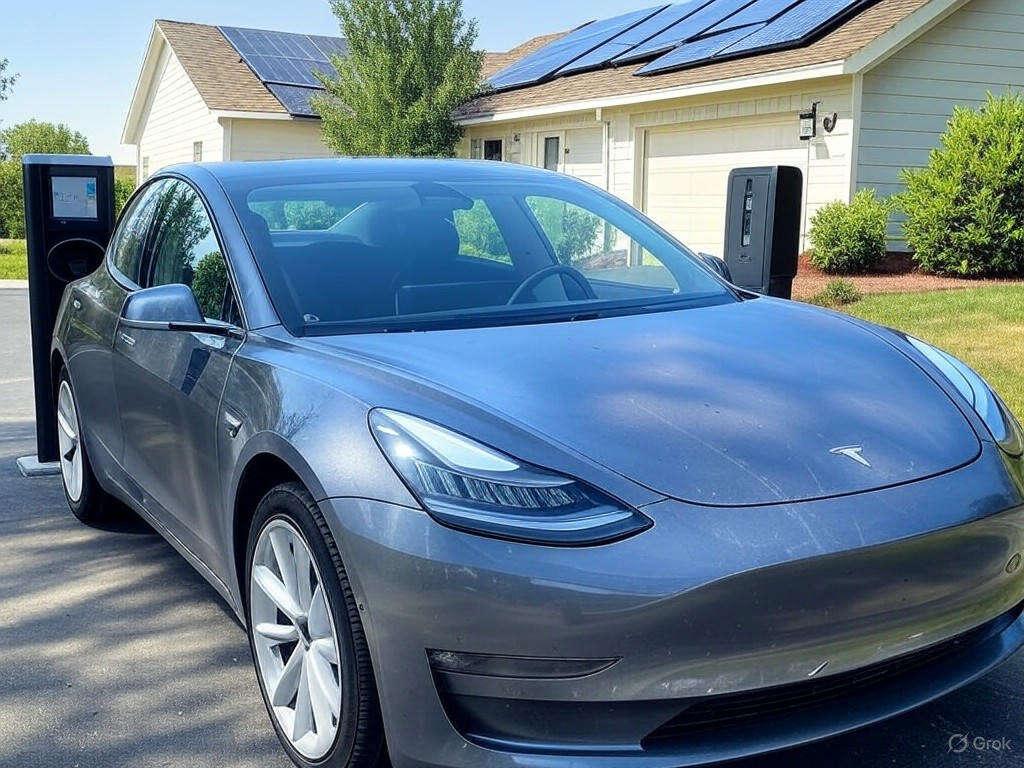
Performance Insights and Real-World Usage: Comparing Technologies and Setups
When charging electric vehicles (EVs) with solar power, the choice of technology and system configuration greatly impacts performance, cost savings, and reliability. Drawing on both extensive testing and real-world user data from 2025, this section evaluates the current landscape of solar EV charging solutions.
Direct DC Charging vs. AC Inverter-Based Systems: Speed and Efficiency
Direct DC solar charging systems represent the forefront of EV charging technology, particularly suited for medium- and heavy-duty vehicles. The National Renewable Energy Laboratory’s (NREL) eCHIP project exemplifies this by developing high-power DC chargers that bypass the traditional AC conversion step. This design improves overall efficiency and reduces system complexity, enabling megawatt-level charging rates essential for electric trucking fleets and commercial operations.
In contrast, residential and smaller commercial solar EV setups predominantly use AC inverter-based systems. These convert the DC electricity generated by solar panels into AC power before feeding it to Level 2 EV chargers. Although this introduces an additional energy conversion step—and some associated efficiency loss—it remains the most cost-effective and practical solution for typical home use.
For example, a 10 kW rooftop solar system paired with a Level 2 AC charger like the Enphase IQ 50 (delivering 9.6 kW) can generate around 40 kWh of usable energy per day under ideal sunlight conditions. This capacity sufficiently covers average daily driving needs for most EV owners.
From a usability standpoint, AC inverter systems offer greater compatibility with existing EV chargers, simpler installation, and broader market availability. Meanwhile, direct DC solar charging, despite its efficiency and rapid charging potential, is still largely confined to experimental or commercial fleet environments requiring specialized infrastructure.
Energy Savings and Grid Interaction: Real-World Numbers
Solar-powered EV charging can substantially reduce grid electricity dependence, translating into significant cost savings. For instance, an average EV consuming approximately 2,000 kWh annually can be largely offset by a 2.35 kWp solar system (around 10 panels) in moderate-sun regions like the UK.
In the U.S., homeowners with 10 kW solar arrays report daily energy yields near 38 kWh, ample for running Level 2 chargers at 32–40 amps comfortably. Financially, a comprehensive solar EV charging setup—including panels, inverter, and a smart Level 2 charger—can cost upwards of $17,000 before incentives. However, federal and state rebates, such as the 30% Residential Clean Energy Credit, significantly reduce this upfront cost.
Over a 25-year period, solar panels can save homeowners roughly $50,000 on electricity, underlining the long-term value of solar EV charging investments.
Smart chargers integrated with energy monitors—like the Emporia Level 2 charger combined with the Vue 3 Home Energy Monitor—optimize solar consumption by prioritizing self-generated power and minimizing grid draw. This intelligent load management not only cuts utility bills but also alleviates peak grid demand and enhances overall system reliability.
Tackling Intermittency: Battery Storage and Bidirectional Charging
Solar energy’s intermittent nature—limited to daylight hours and subject to weather variability—remains the primary practical limitation for solar EV charging. Without energy storage, EV owners must rely on grid electricity during low solar production periods, such as cloudy days or winter months.
Home battery storage solutions, including Tesla Powerwall 3, Panasonic EVERVOLT, and LG Home 8, buffer this variability by storing excess solar energy for use during nighttime or low-sun conditions. These lithium-ion systems, often employing lithium ferro phosphate (LFP) chemistry for enhanced safety and longevity, help push solar-powered EV charging closer to full grid independence.
Bidirectional chargers are an emerging technology that further mitigates solar intermittency challenges. By enabling Vehicle-to-Grid (V2G) and Vehicle-to-Home (V2H) functionality, these chargers allow EV batteries to serve as distributed energy storage assets.
For example, Massachusetts is deploying 100 bidirectional Level 2 chargers in a Vehicle-to-Everything (V2X) pilot program, targeting 1.5 MW of flexible grid capacity by late 2026. On a daily basis, bidirectional charging permits drawing power from the EV battery during peak demand or outages and recharging it when solar generation or low-cost grid power is available. This functionality enhances home energy resilience and can generate additional revenue through grid demand-response participation.
Challenges remain, including limited EV model compatibility with required communication protocols and evolving regulatory frameworks. Nonetheless, early real-world data shows that integrating bidirectional charging with solar arrays offers a robust strategy to maximize economic, environmental, and reliability benefits.
Case Studies: Typical Usage and Cost Outcomes
Seasonal and daily solar production patterns significantly affect home solar EV charging performance. Peak solar irradiation typically reaches about 7.6 kWh/m²/day in late spring and summer, dropping to approximately 3.8 kWh/m²/day during winter.
A typical 10 kW residential system yields around 38 kWh daily, sufficient to fully charge most EVs with 60–75 kWh batteries if charging is scheduled throughout the day. For instance, one project combined a 10.7 kW solar array with a JuiceBox 32 Amp Level 2 charger. After accounting for system losses—including temperature effects, inverter inefficiency, and panel soiling—the usable real-time power was roughly 7.6 kW. This configuration enabled primarily solar-powered EV charging, reducing grid dependency and yielding annual energy cost savings of several hundred dollars.
In the UK, data reveals that EV owners are seven times more likely to have solar panels than non-EV owners, highlighting the growing synergy between solar adoption and EV ownership. Homeowners leveraging time-of-use (TOU) electricity tariffs and smart charging applications can further optimize savings by aligning EV charging with peak solar production hours.
Final Thoughts
-
AC inverter-based solar EV charging remains the most practical and cost-effective solution for most homeowners in 2025, delivering reliable Level 2 charging speeds and meaningful energy cost reductions.
-
Direct DC solar charging is a promising frontier tailored for commercial fleets and ultra-fast charging but is not yet widely adopted at the residential level.
-
Intermittency challenges can be effectively mitigated with home battery storage and bidirectional charging technologies, which also provide grid support and potential financial incentives.
-
Real-world data confirms that a properly sized solar array combined with smart charging technologies yields significant savings and enhances energy independence, making solar EV charging a sound long-term investment.
If you are considering solar-powered EV charging, focus on selecting the right combination of panel capacity, inverter quality, and a smart Level 2 charger. Incorporating battery storage and exploring bidirectional charging options can future-proof your system, maximizing both efficiency and resilience.
Aspect | Direct DC Charging | AC Inverter-Based Systems |
---|---|---|
Target Usage | Medium- and heavy-duty vehicles, commercial fleets | Residential and smaller commercial setups |
Charging Speed | Megawatt-level charging rates | Level 2 charging (up to ~9.6 kW) |
Efficiency | High (bypasses AC conversion) | Lower (additional AC conversion step) |
System Complexity | Reduced complexity | More complex due to inverter |
Installation | Specialized infrastructure needed | Simple and widely available |
Market Availability | Experimental, commercial fleet focused | Broadly available for homes |
Cost | Higher, specialized equipment | More cost-effective for home use |
Metric | Example / Data |
---|---|
Typical Solar System Size | 10 kW rooftop system (approx. 10 panels) |
Daily Usable Energy | ~38-40 kWh (ideal conditions) |
Average EV Annual Consumption | ~2,000 kWh |
Energy Savings Over 25 Years | ~$50,000 |
Upfront Cost (Panels + Inverter + Charger) | ~$17,000 before incentives |
Federal Incentive | 30% Residential Clean Energy Credit |
Level 2 Charger Examples | Enphase IQ 50 (9.6 kW), JuiceBox 32 Amp, Emporia Level 2 |
Battery Storage Examples | Tesla Powerwall 3, Panasonic EVERVOLT, LG Home 8 |
Technology | Description | Benefits | Challenges |
---|---|---|---|
Battery Storage | Stores excess solar energy for use during night or low sun | Mitigates intermittency, enhances grid independence | Cost, space requirements |
Bidirectional Charging | Enables Vehicle-to-Grid (V2G) and Vehicle-to-Home (V2H) functions | Energy resilience, grid support, potential revenue | Limited EV compatibility, evolving regulations |
Parameter | Example/Outcome |
---|---|
Seasonal Solar Irradiance | 7.6 kWh/m²/day (summer), 3.8 kWh/m²/day (winter) |
Usable Power from 10.7 kW System | ~7.6 kW after losses |
Annual Energy Cost Savings | Several hundred dollars |
UK Solar Adoption Among EV Owners | 7x more likely than non-EV owners |
Optimization Method | Time-of-use tariffs and smart charging apps |
Common Challenges and Troubleshooting Your Solar EV Charging System
Common Challenges and Troubleshooting Your Solar EV Charging System
Charging your electric vehicle (EV) with solar power is a smart strategy to reduce reliance on the grid and lower energy costs. However, it comes with technical and practical challenges that can affect the efficiency and reliability of your setup. From hardware mismatches to evolving utility policies, several factors require attention to optimize your solar EV charging system.
This section outlines common issues you may encounter, practical solutions for maintaining peak performance, and guidance on navigating regulatory landscapes to maximize your investment.
Technical Challenges: Insufficient Solar Generation, Inverter Mismatch, and Charger Compatibility
A frequent challenge is insufficient solar generation to fully meet your EV’s charging demands. This typically occurs when the solar array is undersized relative to the EV’s energy consumption. For example, a typical EV in the UK consumes about 2,000 kWh annually, which generally requires a 2.35 kWp solar system to cover charging needs under average sun conditions. Smaller systems or locations with lower solar irradiance will lead to a higher dependency on grid electricity.
Inverter mismatch is another common issue. Solar inverters convert the DC power from solar panels into AC power usable by your home and EV charger. High inverter efficiency—above 95%—is ideal. However, selecting an inverter that is not well-matched to your panel configuration or system size may cause energy losses or unstable output. For instance, string inverters are cost-effective but less adaptable to partial shading or complex roof layouts. Conversely, microinverters or DC optimizers offer better performance in such scenarios by optimizing output at the panel level.
Charger compatibility plays a critical role in harnessing solar power effectively. Not all EV chargers can prioritize solar energy. Smart chargers, such as Enphase’s IQ EV Charger 2 or SolarEdge’s solar-first EV chargers, enable solar power prioritization and intelligent charging schedules based on solar availability. Standard chargers typically draw grid power by default, which diminishes the benefits of your solar system.
Key recommendations:
- Size your solar array to align with your EV’s charging needs, usually 7–12 panels (approximately 3–5 kW) for typical home EV owners.
- Choose high-efficiency inverters compatible with your panel layout; consider microinverters or DC optimizers if shading or panel orientation is a concern.
- Invest in smart EV chargers that support solar-first charging and integrate with home energy management systems to maximize solar self-consumption.
Practical Solutions: Maintenance, Correct Sizing, Software Updates, and Battery Care
Achieving sustained system performance requires regular maintenance and accurate system sizing. Dirty solar panels can reduce energy output by 5–30%, depending on local conditions such as dust, bird droppings, or pollution. Cleaning panels at least twice annually is a practical baseline, with more frequent cleaning advisable in dusty or polluted environments.
Proper system sizing is essential. Oversizing can lead to unnecessary expenses and inverter stress, while undersizing results in insufficient solar energy for EV charging. Calculate your system size based on your EV’s mileage, charging habits, and local solar insolation data. Planning for a 20–30% oversizing buffer can future-proof your system against increased energy needs.
Smart charger firmware updates are often overlooked but crucial. Manufacturers regularly release updates that improve energy management algorithms, grid communication, and solar integration. Stay current by checking your charger’s app or manufacturer website to ensure optimal performance and stability.
On the EV side, battery maintenance improves longevity and charging efficiency. Recommended practices include avoiding routine charging beyond 80%, minimizing fast charging to reduce heat stress, and parking in shaded or temperature-controlled environments. Maintaining battery health is vital since replacement costs can reach 30–40% of the vehicle’s price.
Maintenance checklist:
- Clean solar panels at least twice yearly; consider professional services for thorough cleaning.
- Reevaluate your solar system size if consistent underperformance occurs.
- Regularly update smart charger firmware to benefit from enhancements and bug fixes.
- Follow EV battery care best practices to extend battery life and maintain efficiency.
Monitoring System Health and Managing Energy
Monitoring your solar EV charging system is vital for maximizing uptime and efficiency. Modern solar setups often include real-time monitoring platforms that track energy production, consumption, and charging patterns. Solutions like ELECQ’s Home Energy Management or Wevo Energy’s AI-powered software intelligently balance loads, prioritize solar power usage, and provide alerts for system faults.
Energy management conflicts can arise when multiple high-power appliances operate simultaneously with your EV charger. Implementing smart load balancing and adaptive energy management—using AI or fuzzy logic controllers—helps dynamically allocate power, prevent circuit overloads, and ensure solar energy is optimally utilized.
Navigating Utility Restrictions and Maximizing Net Metering Benefits
Utility policies concerning net metering and solar interconnection can significantly impact the economics of your solar EV charging system. Net metering programs credit customers for excess solar energy exported to the grid, but many utilities are revising these policies.
For example, states like Idaho and North Carolina have transitioned from traditional net metering to time-of-use (TOU) export rates, which offer variable compensation depending on when energy is sent back to the grid. Some states have capped or eliminated net metering programs, although grandfathering provisions often protect existing customers.
Before installation, verify local utility interconnection rules, application processes, and net metering terms. Systems under 15 kW typically qualify for simplified interconnection, while larger arrays may require additional infrastructure such as dedicated communication lines or advanced metering.
Integrating battery storage with your solar panels can mitigate export limitations by storing excess solar energy for later use, increasing self-consumption and reducing grid dependency.
Action steps:
- Research your local net metering policies and stay informed about upcoming changes.
- Consider adding home battery storage to maximize solar utilization and navigate export restrictions.
- Partner with experienced installers familiar with local utility requirements to streamline approval and maximize incentives.
Solar-powered EV charging offers a compelling blend of cost savings, environmental benefits, and energy independence. By proactively addressing technical challenges like inverter mismatch and charger compatibility, maintaining your system diligently, and staying current with utility policies, you can ensure a reliable and efficient solar EV charging experience for years to come.
Category | Common Issues | Practical Solutions / Recommendations |
---|---|---|
Technical Challenges | Insufficient solar generation Inverter mismatch Charger compatibility | – Size solar array to match EV needs (7–12 panels, approx. 3–5 kW) – Use high-efficiency inverters & consider microinverters/DC optimizers – Invest in smart EV chargers supporting solar-first charging |
Practical Solutions | Dirty solar panels reducing output Incorrect system sizing Outdated smart charger firmware EV battery degradation | – Clean panels at least twice yearly – Reevaluate system sizing with 20–30% oversize buffer – Update charger firmware regularly – Follow EV battery care best practices (limit charge to 80%, reduce fast charging, park shaded) |
Monitoring & Energy Management | Energy production/consumption imbalance Load conflicts with other appliances | – Use real-time monitoring platforms – Implement smart load balancing and adaptive energy management (AI or fuzzy logic) |
Utility Restrictions & Net Metering | Changing net metering policies Export limits and rate variability Interconnection requirements | – Research local net metering and utility rules – Consider home battery storage to increase self-consumption – Work with experienced installers for approvals and incentives |
Advanced Optimization and Future-Proofing Your Solar EV Charging Setup
Advanced Optimization and Future-Proofing Your Solar EV Charging Setup
When designing a solar-powered EV charging system, it’s essential to think beyond the immediate installation. The energy technology landscape evolves rapidly, and integrating smart energy management, thoughtfully oversizing your system, and preparing for emerging standards like vehicle-to-grid (V2G) technology will maximize both your financial investment and environmental benefits over the solar panel lifespan of 25 to 30 years.
Smart Home Integration and AI-Driven Energy Management
Modern solar EV charging setups are increasingly embracing smart home integration and AI-driven energy management to optimize solar power usage and reduce grid dependence.
Level 2 EV chargers such as the Emporia Pro (48A) and Wallbox Pulsar Plus (up to 48A, 11.5 kW) come with built-in solar management features. These chargers intelligently schedule charging sessions to prioritize self-consumption of solar energy, lowering electricity costs and minimizing grid electricity use. For instance, the Wallbox Pulsar Plus paired with its dedicated energy meter allows granular control via its app, dynamically adjusting charging rates based on real-time solar output and household loads.
AI-powered energy management systems take optimization further by learning your household’s consumption patterns alongside local weather forecasts. According to Illinois Renewables, such AI-driven solutions can reduce home energy bills by approximately 30% by adapting charging schedules in real time to maximize solar utilization and avoid unnecessary grid draw.
Commercial-grade solutions like SolarEdge’s EV charger have demonstrated up to 70% reductions in fleet charging costs by prioritizing solar self-consumption. While currently targeted at commercial fleets, these advanced technologies are expected to become widely available for residential use, signaling a future where AI-managed solar EV charging is standard.
Oversizing Solar Systems for Future EVs and Household Loads
Planning your solar array size with future growth in mind safeguards your investment and prevents costly system upgrades.
Typical residential solar systems range from 18 to 26 panels (approximately 6 to 9 kW) to cover average household electricity needs. Adding an EV charger increases demand by 1.2 kW (Level 1) up to 11.5 kW (Level 2). To accommodate anticipated EV adoption or increased home energy use—such as adding batteries or new appliances—oversizing your system by 20–30% beyond current consumption is prudent.
Contrary to some misconceptions, most U.S. utilities do not impose strict limits on solar system oversizing. However, it’s important to verify local interconnection policies as some utilities may cap allowable system capacity or impose specific requirements.
Oversizing also boosts self-consumption, especially when paired with home battery storage solutions like the Tesla Powerwall 3 or Enphase IQ8. These batteries provide 8 to 24 hours of backup and load shifting, enabling excess solar energy to be stored and used for nighttime EV charging or during grid outages.
Roof space and panel orientation remain critical. A 10 kW system typically requires 360 to 510 square feet of well-oriented roof area (preferably true south with optimal tilt). Adopting a modular design approach allows for adding panels or batteries later, supporting scalability and evolving household energy needs.
Vehicle-to-Grid (V2G) Technology and Scalable Equipment
V2G technology is an emerging advancement that transforms your EV battery into a mobile energy storage unit capable of returning electricity to your home or the grid.
Though still gaining traction, V2G solutions like Virta’s maintain EV battery charge between 70% and 90% while enabling energy to flow back to the grid during peak demand periods. This supports grid stability and offers potential revenue streams for EV owners through demand response programs. Industry forecasts suggest that by 2030, up to 250 million EVs may participate in V2G, highlighting its transformative potential.
Eaton is advancing vehicle-to-home (V2H) technology, allowing EV batteries to bridge power outages for 10 to 20 hours via bi-directional charging. These solutions require compatible hardware and compliance with evolving standards such as UL 3141, which certifies power control systems for safety and scalability.
When selecting equipment, prioritize chargers and inverters that are V2G-ready or support future firmware upgrades to ensure compatibility. Companies like Delta Electronics are developing scalable, megawatt-level charging infrastructure for fleets, reflecting industry momentum toward modular and expandable systems.
Maximizing ROI and Environmental Impact Over Solar Panel Lifespan
Solar panels typically last 25 to 30 years, retaining around 80% of their original efficiency after 25 years. Modern monocrystalline panels offer an energy payback period of only 1 to 4 years, meaning they generate several times the energy required for their manufacture over their lifespan.
This durability translates into decades of carbon-free electricity, significantly lowering your household’s carbon footprint. Advances in manufacturing sustainability and recycling technologies have improved recovery rates, with over 95% of some panel components now recyclable—making solar a responsible environmental choice.
To maximize your return on investment:
- Combine solar panels with smart energy management and home battery storage to increase solar self-consumption and reduce reliance on grid electricity.
- Oversize your solar system moderately (20–30%) to accommodate future EVs or increased household loads, avoiding costly upgrades.
- Choose equipment backed by strong warranties (typically 15 to 25 years) and reputable manufacturer support.
- Use smart sensors and monitoring apps to track performance, detect degradation early, and ensure consistent system output.
Drawing from industry data and user experiences, solar EV charging setups that incorporate AI-driven management and scalable designs consistently deliver superior long-term value and satisfaction. By planning for anticipated technology advances and evolving energy needs today, your solar EV charging system will remain efficient, adaptable, and environmentally impactful for decades to come.
Category | Details |
---|---|
Smart Home Integration and AI-Driven Energy Management | Level 2 chargers (Emporia Pro 48A, Wallbox Pulsar Plus up to 48A/11.5 kW) with solar management features; AI systems reduce energy bills ~30%; Commercial SolarEdge EV charger reduces fleet costs by up to 70% |
Oversizing Solar Systems | Residential systems: 18-26 panels (6-9 kW); Oversize by 20-30% for future EVs/loads; Utilities vary on oversizing limits; Modular design recommended; Roof space 360-510 sq ft for 10 kW system |
Battery Storage | Tesla Powerwall 3, Enphase IQ8 provide 8-24 hours backup/load shifting; Enables nighttime EV charging and grid outage resilience |
Vehicle-to-Grid (V2G) and Vehicle-to-Home (V2H) Technology | V2G tech (e.g. Virta) maintains 70-90% battery charge, supports grid demand response; Eaton V2H offers 10-20 hours backup; Requires V2G-ready chargers/inverters and compliance with standards (UL 3141) |
Equipment Recommendations | Choose V2G-ready or upgradeable chargers/inverters; Scalable megawatt charging infrastructure by Delta Electronics |
Solar Panel Lifespan and Environmental Impact | 25-30 years lifespan; ~80% efficiency at 25 years; Energy payback 1-4 years; >95% recyclability for some components; Significant carbon footprint reduction |
Maximizing ROI | Combine panels with smart management and battery storage; Moderate oversizing (20-30%); Select strong warranties (15-25 years); Use sensors and monitoring apps |