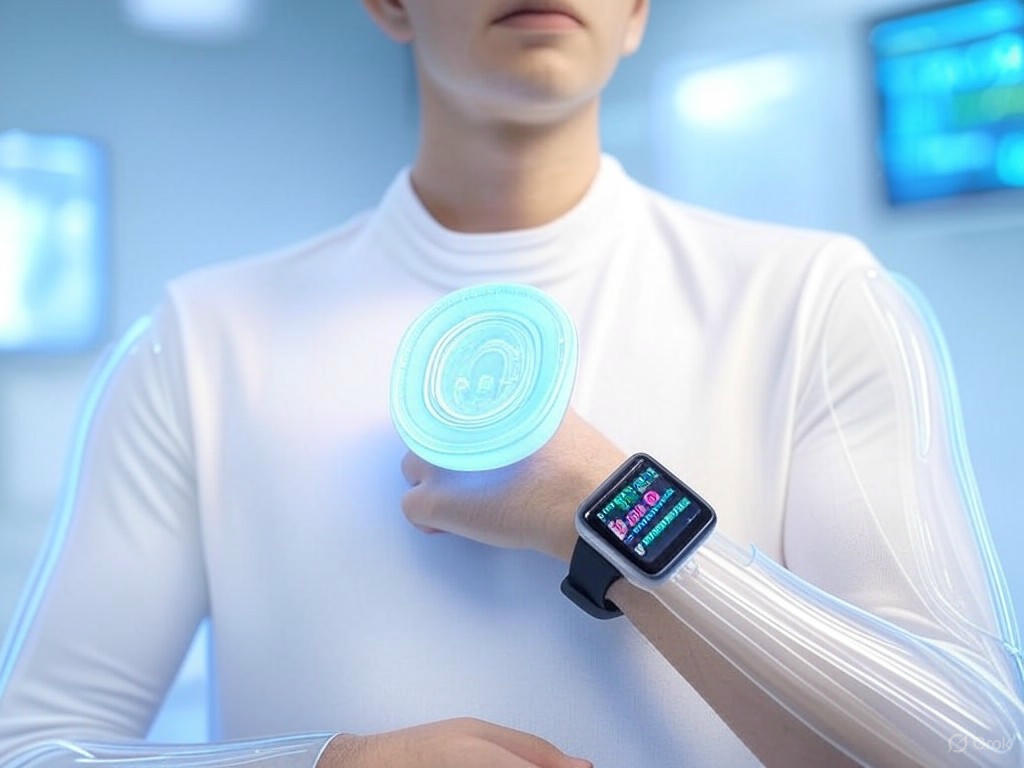
Health Implantables vs Wearables: Real Insights on Future Monitoring
- Introduction: Defining the Landscape of Health Implantables vs Wearables
- Introduction: Defining the Landscape of Health Implantables vs Wearables
- Defining Implantables and Wearables
- Market Trends and Projected Growth
- Analytical Framework for This Article
- Technical Specifications and Innovations in Implantable and Wearable Devices
- Technical Specifications and Innovations in Implantable and Wearable Devices
- Hardware Components: Sensors, Power Sources, and Form Factors
- Communication Protocols: Connectivity with Security and Low Power
- Software Capabilities: AI Integration, Data Processing, and Interoperability
- Cutting-Edge Innovations: Graphene Sensors and Biofilm Energy Generation
- Performance Metrics and Benchmarking: Accuracy, Latency, and Reliability
- Performance Metrics and Benchmarking: Accuracy, Latency, and Reliability
- Accuracy of Vital Sign Monitoring: Implantables vs. Wearables
- Latency and Real-Time Responsiveness
- Reliability: Battery Life, Sensor Stability, and Durability
- Data Integrity and Error Rates: Clinical vs. Consumer Devices
- User Experience and Practical Implications in Daily Life
- User Experience and Practical Implications in Daily Life
- Comfort, Maintenance, Invasiveness, and User Compliance
- Data Accessibility and Interpretability from the User Perspective
- Psychological and Social Impacts: Visible Wearables Versus Invisible Implantables
- Real-World Use Cases
- Comparative Analysis: Health Implantables vs Wearables in Monitoring Capability and Application Scope
- Comparative Analysis: Health Implantables vs Wearables in Monitoring Capability and Application Scope
- Monitoring Range and Data Granularity
- Clinical Relevance and Suitability Across Conditions
- Integration with Telemedicine and Electronic Health Records
- Regulatory and Privacy Challenges
- Summary
- Strengths and Limitations with Technical and Practical Explanations
- Strengths and Limitations with Technical and Practical Explanations
- Implantables: Superior Data Fidelity with Intrinsic Challenges
- Wearables: Versatile, User-Friendly Devices with Technical Trade-Offs
- Areas Requiring Further Innovation
- Summary
- Final Verdict and Future Outlook: Evidence-Based Recommendations for Users and Developers
- Final Verdict and Future Outlook: Evidence-Based Recommendations for Users and Developers
- Performance and User Experience: Implantables vs. Wearables
- Practical Guidance: When to Choose Which?
- Emerging Innovations: What’s Next?
- Privacy, Ethics, and Regulatory Landscape
- Market Evolution and Healthcare Integration
- Key Takeaways
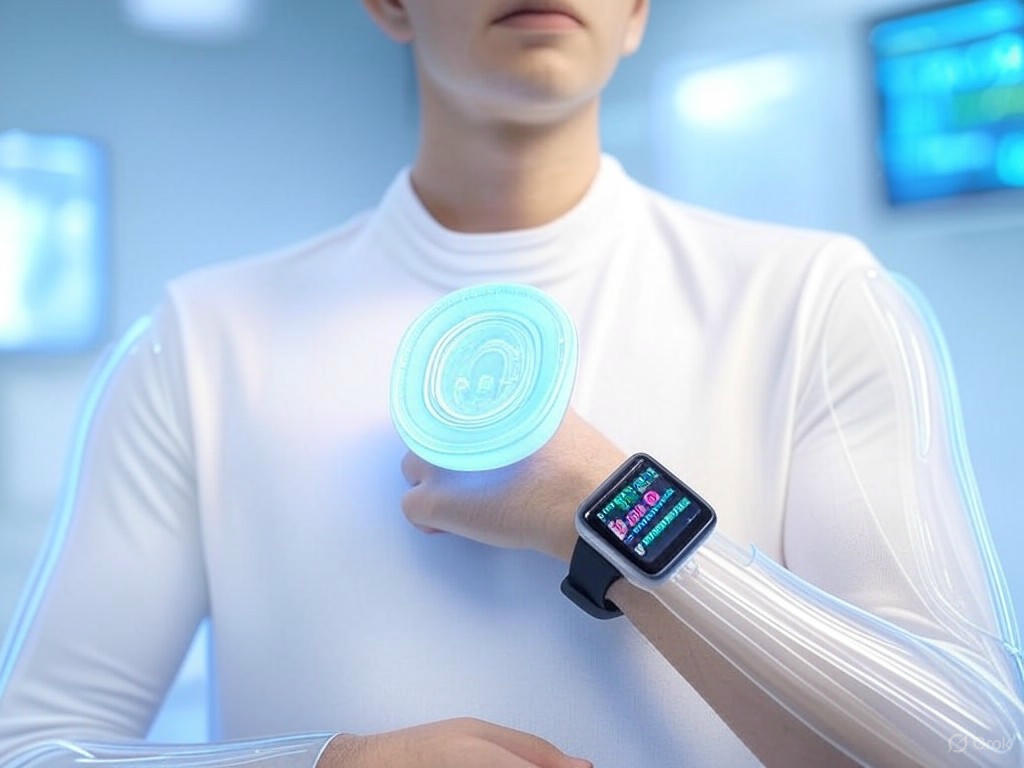
Introduction: Defining the Landscape of Health Implantables vs Wearables
Introduction: Defining the Landscape of Health Implantables vs Wearables
Continuous health monitoring has become an indispensable element in modern medicine and consumer health management. Traditional hospital protocols often depend on periodic “spot-checking” of vital signs every four to eight hours, creating substantial gaps in real-time patient data. For instance, U.S. hospitals report approximately 1,000 daily deaths from preventable adverse events, many of which could be mitigated through continuous monitoring technologies. Amid growing nurse shortages and an aging population—particularly the baby boomer generation—technology-driven patient monitoring is transitioning from optional to essential. Continuous monitoring solutions empower healthcare teams to track critical parameters such as heart rate, respiratory rate, and movement in real time, thereby improving clinical outcomes and alleviating healthcare workloads.
Defining Implantables and Wearables
Health implantables and wearables share the overarching objective of delivering personalized, real-time health data, yet they differ fundamentally in form factor, deployment, and clinical application.
-
Health Implantables are devices surgically or minimally invasively inserted within the body. Examples include pacemakers, implantable cardioverter defibrillators (ICDs), cochlear implants, and orthopedic implants like artificial joints. These devices often perform critical therapeutic functions—regulating heart rhythms or assisting organ function—and adhere to stringent regulatory approvals such as FDA PMA or 510(k) clearances. Modern implantables increasingly feature cloud connectivity, artificial intelligence (AI), and Internet of Things (IoT) integration, enhancing their role in precision medicine and chronic disease management.
-
Wearables are externally worn electronic devices equipped with sensors that continuously track physiological and biometric data. This category encompasses smartwatches, fitness bands, continuous glucose monitors (CGMs), and emerging AI-powered hearing aids. Wearables provide non-invasive, real-time monitoring designed for convenience and daily use, often synchronizing with smartphones and telemedicine platforms. While traditionally focused on health maintenance, fitness, and early disease detection, wearables are rapidly expanding into medical-grade applications.
Both implantables and wearables form foundational pillars of the evolving personalized medicine ecosystem, enabling tailored treatments informed by genetic, lifestyle, and environmental factors. They support remote patient monitoring, reduce the necessity of hospital visits, and facilitate timely clinical interventions.
Market Trends and Projected Growth
The health implantable and wearable markets exhibit robust growth trajectories, albeit with differing scales and dynamics:
-
The implantable medical device market was valued at approximately $83 billion in 2024, projected to reach nearly $126 billion by 2030, reflecting a compound annual growth rate (CAGR) of about 7.2%. Key growth drivers include an aging demographic, rising prevalence of chronic diseases, and advancements in biocompatible materials and minimally invasive surgical techniques. Nonetheless, supply chain complexities and regulatory challenges pose ongoing hurdles.
-
The wearable medical device market is expanding at an even faster pace, with an estimated $54 billion valuation in 2025 and projections exceeding $427 billion by 2034. This high single- to low double-digit CAGR is fueled by increasing consumer health awareness, the growing incidence of chronic conditions like diabetes and cardiovascular diseases, and wider adoption in preventive care. North America currently leads in market share, while Asia-Pacific exhibits accelerated growth owing to supportive policies and escalating healthcare expenditures.
These trends underscore a decisive shift toward continuous, data-driven, and patient-centric healthcare models powered by connected devices.
Analytical Framework for This Article
To deliver a comprehensive and balanced analysis, this article evaluates health implantables and wearables through three critical perspectives:
-
Technical Specifications: Assessing device accuracy, sensor sophistication, connectivity protocols, battery life, invasiveness, and regulatory compliance. This foundation is essential for evaluating clinical efficacy and practical deployment.
-
User Experience: Examining real-world usability, comfort, integration into daily life, and accessibility of data. Devices excelling technically but lacking user adherence or convenience risk limited impact.
-
Innovation and Future Potential: Highlighting genuine technological breakthroughs such as AI integration, biosensor advancements, energy harvesting innovations, and personalized treatment capabilities. This includes candid discussion of limitations and challenges, including privacy concerns and cost barriers.
Through this tripartite framework, the article aims to distinguish marketing hyperbole from substantive value, guiding clinicians, patients, and consumers in navigating the rapidly evolving health monitoring landscape.
In summary, continuous health monitoring is transitioning from episodic assessments to seamless, 24/7 data streams enabled by implantables and wearables. These device categories complement each other: implantables provide critical, life-sustaining functions with clinical-grade precision, while wearables facilitate proactive health management and early intervention through accessible, non-invasive monitoring. With substantial market growth and accelerating innovation, a nuanced understanding of these technologies’ capabilities and real-world performance is more vital than ever.
Aspect | Health Implantables | Wearables |
---|---|---|
Form Factor | Surgically or minimally invasively inserted within the body | Externally worn electronic devices |
Examples | Pacemakers, ICDs, cochlear implants, orthopedic implants | Smartwatches, fitness bands, continuous glucose monitors, AI-powered hearing aids |
Primary Functions | Critical therapeutic functions (e.g., regulating heart rhythm, assisting organ function) | Non-invasive, real-time monitoring for health maintenance, fitness, early disease detection |
Regulatory Approvals | FDA PMA or 510(k) clearances, stringent regulatory standards | Increasingly medical-grade, but traditionally consumer-focused |
Technology Integration | Cloud connectivity, AI, IoT integration | Synchronization with smartphones, telemedicine platforms, AI features |
Market Size (2024/2025) | Approximately $83 billion (2024) | Approximately $54 billion (2025) |
Projected Market Size | Nearly $126 billion by 2030 (CAGR ~7.2%) | Over $427 billion by 2034 (high single- to low double-digit CAGR) |
Growth Drivers | Aging demographic, chronic diseases, biocompatible materials, minimally invasive techniques | Consumer health awareness, chronic conditions prevalence, preventive care adoption |
Challenges | Supply chain complexities, regulatory hurdles | Privacy concerns, cost barriers, user adherence |
User Experience | Implantation required, clinical-grade precision | Non-invasive, designed for comfort and daily use |
Role in Healthcare | Life-sustaining, clinical interventions | Proactive health management, early intervention |
Technical Specifications and Innovations in Implantable and Wearable Devices
Technical Specifications and Innovations in Implantable and Wearable Devices
Hardware Components: Sensors, Power Sources, and Form Factors
Health implantables and wearables both depend on sophisticated sensors to capture accurate physiological data. Key sensor types include photoplethysmography (PPG) for blood volume and oxygen saturation, electrocardiogram (ECG) sensors for heart rhythm monitoring, and accelerometers that track motion and activity levels. Notably, recent advances in 2025 have enabled the miniaturization of PPG and ECG sensors tailored for implantables, delivering enhanced accuracy and long-term stability crucial for clinical-grade monitoring [Sources 0, 2].
Wearable devices leverage flexible electronics and user-centric form factors such as smartwatches, biopatches, and smart glasses. These designs emphasize comfort and daily wearability, facilitating high user adherence. Conversely, implantables require a delicate balance between extreme miniaturization and biocompatibility. They must be sufficiently small to minimize tissue irritation and immune response, yet robust enough to integrate complex electronics and power management. Innovations in ultra-fine wiring and biocompatible nanomaterials—including antibacterial coatings and tissue-growth enhancers—are advancing implantable device safety and longevity [Sources 6, 9].
Energy autonomy is a critical frontier connecting both device classes. Beyond conventional batteries, innovative energy-harvesting methods exploit the body’s own energy sources—such as mechanical motion, thermal gradients, and biochemical reactions—to power devices sustainably. A prominent breakthrough is the dual-energy harvesting implant that simultaneously converts magnetic field and ultrasound energy, generating up to three times the power of existing devices. This technology not only extends operational lifespan but also supports millimeter-scale, potentially battery-free implants, enhancing scalability and patient comfort [Sources 4, 7, 5].
Communication Protocols: Connectivity with Security and Low Power
Bluetooth Low Energy (BLE) remains the cornerstone wireless communication protocol for both implantables and wearables, favored for its low power consumption and reliable data throughput. Modern BLE implementations incorporate robust security measures such as LE Secure Connections, MAC address randomization to combat tracking, and whitelist-based authentication, ensuring privacy and data integrity in sensitive health monitoring applications [Sources 0, 2].
For implantables, where power and size constraints are stringent, emerging miniature chipsets like Silicon Labs’ BG29 are pivotal in reducing energy consumption without compromising functionality. These chipsets enable continuous, secure communication with external devices such as smartphones or dedicated readers, which is essential for persistent patient monitoring [Source 1].
Near Field Communication (NFC) is gaining traction, particularly for ultra-short-range, secure interactions in both implantables and wearables. Its capability for two-way communication within a few centimeters makes it ideal for applications such as updating electronic health records (EHRs), modifying device settings, or enabling contactless payments without exposing data to broader networks. The NFC healthcare market is projected to reach $425 million by 2030, underscoring its growing role in connected medical ecosystems [Sources 5, 7].
Additional protocols like NB-IoT, Sigfox, and Thread cater to specific use cases involving low-power wide-area networks (LPWANs) or mesh networking. However, BLE and NFC remain the primary communication pillars in current medical device connectivity [Source 11].
Software Capabilities: AI Integration, Data Processing, and Interoperability
Software advances distinguish implantables and wearables in their capacity to translate raw sensor data into actionable health insights. On-device processing has evolved from basic signal capture to sophisticated AI-driven analytics that enhance accuracy, enable early disease detection, and provide personalized monitoring recommendations. For wearables, AI algorithms help compensate for inherent sensor limitations, improving clinical relevance and user engagement [Sources 0, 1].
Interoperability remains a vital challenge and opportunity. Despite over 90% of healthcare institutions employing multiple medical devices, only about 30% achieve full integration with electronic health records (EHRs). Efforts to standardize data exchange via frameworks such as the US Core Data for Interoperability (USCDI) and ANSI/AAMI/UL 2800-1 are facilitating secure, seamless sharing of device data across platforms and providers, thereby enhancing coordinated patient care [Sources 4, 7].
The Internet of Medical Things (IoMT) ecosystem is expanding rapidly, linking implantables and wearables to cloud-based services where AI-powered analytics synthesize data streams into predictive health models. This integration promises improved patient outcomes, reduced hospitalizations, and cost savings through remote monitoring and proactive interventions [Sources 5, 3].
Cutting-Edge Innovations: Graphene Sensors and Biofilm Energy Generation
Emerging materials and energy technologies are pushing the boundaries of health monitoring devices. Graphene-based electrochemical biosensors exemplify this trend, leveraging graphene’s exceptional electrical conductivity—surpassing copper—and mechanical strength—exceeding diamond—to provide ultra-sensitive detection capabilities. These sensors are being integrated into wearable formats with promising potential for future implantable applications. Additionally, graphene aerogel and hydrogel fibers offer flexible, energy-storing electrodes that may enhance device longevity and reliability [Sources 8, 11].
Another pioneering approach is biofilm energy generation, which harnesses the metabolic activity of biofilms to produce electrical energy internally within implantable devices. This bio-electrochemical method could enable self-sustaining power sources, mitigating limitations of traditional batteries and external energy harvesters. Although in early research stages, biofilm energy generation holds transformative potential for extending implantable device autonomy and reducing maintenance interventions [Sources 9, 10].
In summary, the convergence between implantable and wearable health devices is evident in their advancing sensor sophistication, energy autonomy, and secure connectivity. Implantables excel in clinical-grade accuracy, biocompatible materials, and breakthrough energy harvesting technologies, while wearables lead in ergonomic design, user-friendly interfaces, and versatile wireless communications. Software innovations—especially in AI integration and interoperability—are critical to maximizing the clinical and personal value of these devices. Looking forward, material science breakthroughs like graphene sensors and novel power sources such as biofilm energy generation are set to redefine the capabilities and impact of continuous health monitoring technologies.
Aspect | Implantable Devices | Wearable Devices |
---|---|---|
Key Sensors | Miniaturized PPG and ECG sensors with clinical-grade accuracy | PPG, ECG, accelerometers with flexible electronics |
Form Factors | Extremely miniaturized, biocompatible, antibacterial coatings, tissue-growth enhancers | Smartwatches, biopatches, smart glasses; user-centric, comfortable |
Power Sources | Dual-energy harvesting (magnetic + ultrasound), biofilm energy generation (early stage) | Conventional batteries, body energy harvesting (motion, thermal, biochemical) |
Communication Protocols | Bluetooth Low Energy (BLE) with low power chipsets (e.g., Silicon Labs BG29), NFC for ultra-short range secure communication | Bluetooth Low Energy (BLE), NFC, flexible wireless protocols |
Security Features | LE Secure Connections, MAC address randomization, whitelist authentication | LE Secure Connections, MAC address randomization, whitelist authentication |
Software Capabilities | AI-driven analytics for clinical-grade monitoring, on-device processing, interoperability with EHRs | AI algorithms to compensate sensor limitations, user engagement, interoperability efforts ongoing |
Interoperability | Partial integration with EHRs (~30%), standards USCDI, ANSI/AAMI/UL 2800-1 applied | Partial integration with EHRs (~30%), standards USCDI, ANSI/AAMI/UL 2800-1 applied |
Innovations in Materials | Biocompatible nanomaterials, antibacterial coatings, graphene sensors potential implantable use | Graphene-based electrochemical biosensors, graphene aerogel/hydrogel electrodes |
Energy Autonomy | Battery-free potential, dual-energy harvesting, biofilm energy generation (research stage) | Battery powered and body energy harvesting methods |
Communication Range | Short range (BLE, NFC), designed for continuous secure communication with external devices | Short range (BLE, NFC), designed for daily use and connectivity with smartphones |
Performance Metrics and Benchmarking: Accuracy, Latency, and Reliability
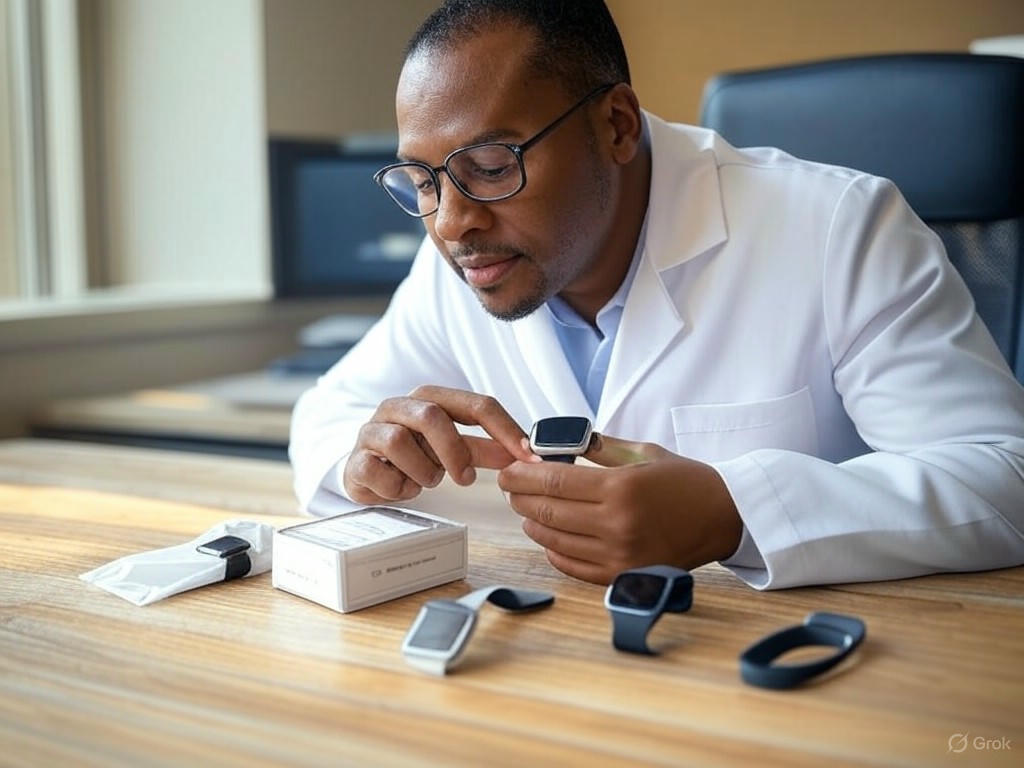
Performance Metrics and Benchmarking: Accuracy, Latency, and Reliability
When evaluating health implantables versus consumer wearables such as the Apple Watch and Fitbit Versa, significant distinctions in accuracy, latency, and reliability emerge. These differences are crucial for patients, clinicians, and consumers who depend on continuous health monitoring for decision-making and wellbeing.
Accuracy of Vital Sign Monitoring: Implantables vs. Wearables
Implantable devices, notably pacemakers and implantable cardioverter-defibrillators (ICDs), represent the clinical gold standard for accuracy. Embedded directly within cardiac tissue, these devices measure electrical signals with exceptional precision, supporting critical therapeutic functions. Their sensor performance remains stable over many years, a necessity for managing chronic conditions effectively.
Key attributes of implantables include:
- Clinical-grade precision: Error rates are minimal due to rigorous FDA PMA and 510(k) regulatory standards.
- Advanced signal processing: For example, modern pacemakers utilize sophisticated QRS complex filtering.
- Robust data security: Hardware-level encryption ensures data integrity during transmission.
- Longevity: Implantable batteries typically last 7 to 12 years, minimizing the need for replacement surgeries.
In contrast, consumer wearables offer impressive but comparatively less precise vital sign monitoring. The Apple Watch, a market leader in smartwatches, uses photoplethysmography (PPG) sensors to estimate heart rate with an accuracy of ±3–5 beats per minute (BPM) at rest. During intense exercise, accuracy may decrease to ±5–10 BPM. A meta-analysis covering 56 studies reported a mean bias of just -0.12 BPM for the Apple Watch, underscoring its reliability as a consumer-grade device. However, the latest Apple Watch Series 10 has encountered critiques due to erratic heart rate spikes during workouts, occasionally exceeding 150 BPM early in exercise sessions, which can undermine confidence in its real-time data.
Fitbit devices, including the Versa and Charge 6 series, excel in heart rate and oxygen saturation (SpO2) tracking, with battery life spanning 5 to 6 days. However, Fitbit currently lacks a fully validated blood pressure monitoring system. Its ongoing development of a Pulse Arrival Time (PAT) method—combining PPG and force sensors—promises improved accuracy in future models but has yet to reach commercial readiness.
Compared to implantables, wearables face inherent challenges such as:
- Sensor placement: Optical sensors are susceptible to skin tone variations, tattoos, and motion artifacts.
- Signal noise: Movement and environmental factors can degrade sensor accuracy.
- Limited clinical validation: Consumer devices prioritize usability and convenience over clinical-grade precision.
Latency and Real-Time Responsiveness
Low latency in data transmission is vital for timely clinical interventions and user engagement. Implantable devices employ secure, low-latency wireless protocols or direct telemetry systems that enable near-instantaneous data transfer to healthcare providers. For instance, ICDs report arrhythmia alerts, battery status, and device integrity with minimal delay, facilitating prompt medical responses.
Consumer wearables, such as the Apple Watch and Fitbit Versa, rely on Bluetooth and Wi-Fi connectivity to sync data with smartphones or cloud services. This approach introduces variable latency influenced by network conditions, software updates, and power-saving strategies. Delays can range from milliseconds to several seconds, particularly during real-time workout monitoring or health events.
Notable impacts of latency in wearables include:
- User engagement: Studies indicate that a 100-millisecond increase in latency can reduce user engagement by 7%.
- Data freshness: Real-time physiological changes may be reported with delays of a few seconds.
- Battery conservation: Sync intervals are often optimized to preserve battery life, especially in Fitbit devices with multi-day endurance.
Fitbit’s emphasis on extended battery life necessitates balancing data transmission frequency against responsiveness. Consequently, while Fitbit wearables perform well for daily wellness tracking, they are less suitable for time-critical health alerts compared to implantables.
Reliability: Battery Life, Sensor Stability, and Durability
Battery longevity is a distinguishing factor between implantables and wearables. Implantable devices are engineered for long-term operation, with battery life spanning 7 to 12 years, depending on usage and device type. Battery replacement involves surgical procedures, underscoring the importance of low power consumption and efficient pacing algorithms.
Wearables require frequent recharging, with typical runtimes as follows:
- Apple Watch models: Approximately 18 to 24 hours per charge.
- Fitbit Versa and Charge series: Approximately 5 to 6 days per charge.
While wearables’ battery life is sufficient for daily use, inconsistent charging habits may cause data gaps. Features such as Apple’s Low Power Mode can extend battery duration by disabling background sensor readings and always-on displays, though at the cost of reduced data granularity and responsiveness.
Sensor stability is another key reliability metric. Implantables maintain consistent, high-fidelity readings unaffected by external factors like motion, skin tone, or environmental conditions. In contrast, wearables’ optical sensors can be disrupted by vigorous activity, darker skin pigmentation, or poor sensor contact. For example, the Apple Watch’s heart rate accuracy deteriorates during intense exercise, and Fitbit devices exhibit similar sensitivity to movement artifacts.
Durability considerations differ markedly:
- Implantables: Protected within the body, these devices withstand physiological conditions and conform to strict biocompatibility standards.
- Wearables: Exposed to impacts, sweat, moisture, and environmental wear, which may degrade sensor performance over time. High-end models like the Apple Watch Ultra 2 and Samsung Galaxy Watch Ultra feature sapphire crystal glass and 10 ATM water resistance (up to 100 meters) to enhance durability.
Data Integrity and Error Rates: Clinical vs. Consumer Devices
Data integrity is paramount for clinical decision-making. Implantables leverage encrypted firmware, hardware signatures, and validated sensor algorithms to minimize error rates. Regulatory agencies such as Health Canada and the U.S. FDA strictly oversee these devices, ensuring robust, continuous data streams for life-critical applications.
Consumer wearables, despite advances, still contend with higher error margins and data noise. Factors contributing to this include:
- Non-clinical design priorities: Emphasis on user-friendliness and broad functionality over stringent accuracy.
- Signal artifacts: Motion, poor sensor contact, and environmental interference.
- Software updates: Changes in firmware or hardware, as seen in Apple Watch Series 10, can introduce temporary regressions in sensor accuracy.
Leading products illustrate this contrast:
- Apple Watch Series 10: Recognized for its comprehensive health monitoring ecosystem but criticized for workout heart rate anomalies.
- Fitbit Charge 6 and Versa: Offer stable heart rate and SpO2 tracking but lack validated blood pressure measurement.
- Implantable pacemakers and ICDs: Deliver precise, continuous ECG data with minimal error, integral for arrhythmia detection and therapy.
In summary, implantable health devices provide unmatched accuracy, near-zero latency, and exceptional reliability essential for critical medical monitoring, albeit with invasiveness and surgical risks. Wearables offer accessible, non-invasive health tracking with good accuracy at rest, moderate latency, and convenient battery life, though their reliability diminishes during intense activity and their clinical precision remains limited for certain parameters like blood pressure.
As the healthcare landscape evolves, implantables and wearables will continue to coexist—implantables reserved for critical clinical needs and wearables serving as everyday health companions. Innovations in AI, sensor miniaturization, and interoperability promise to further bridge the gap between these domains, enhancing personalized medicine and proactive health management.
Metric | Health Implantables | Consumer Wearables (Apple Watch, Fitbit) |
---|---|---|
Accuracy |
|
|
Latency |
|
|
Battery Life |
|
|
Reliability (Sensor Stability & Durability) |
|
|
Data Integrity & Error Rates |
|
|
User Experience and Practical Implications in Daily Life
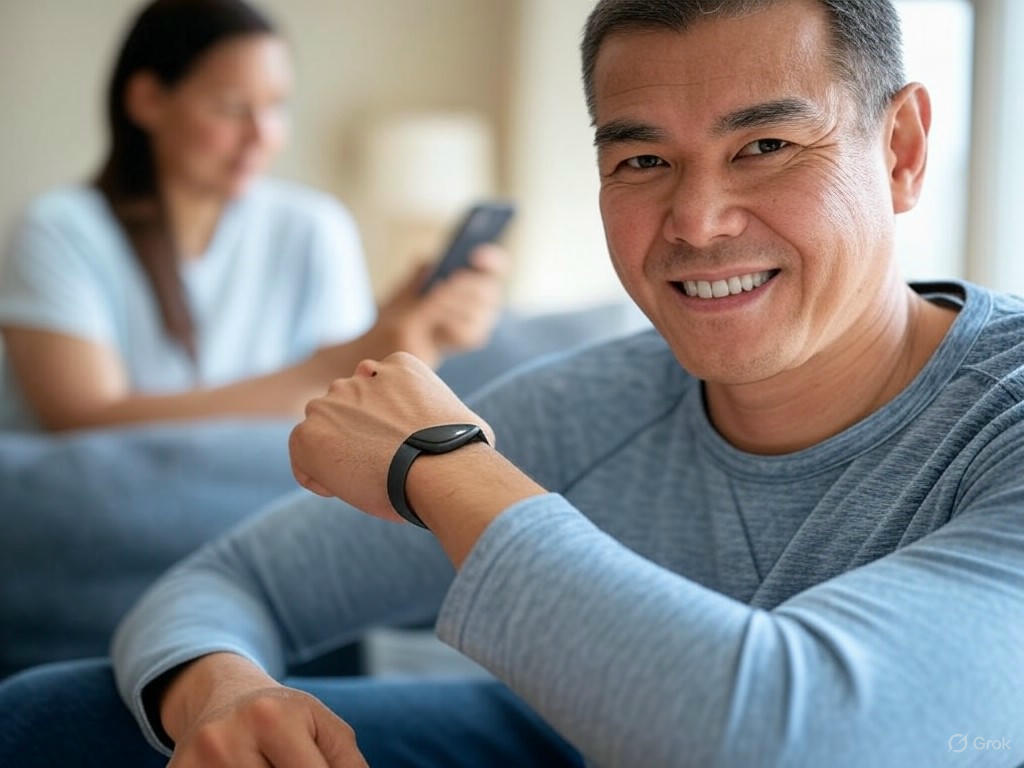
User Experience and Practical Implications in Daily Life
The choice between health implantables and wearables for monitoring hinges significantly on usability factors that influence everyday life. Comfort, maintenance requirements, invasiveness, and psychological and social impacts all play critical roles in user acceptance and compliance. Understanding these distinctions is essential for patients, clinicians, and healthcare providers making informed decisions.
Comfort, Maintenance, Invasiveness, and User Compliance
Wearables excel in straightforward usability due to their noninvasive, plug-and-play nature. Devices such as smartwatches, fitness bands, or rings can be set up within minutes by the user, requiring no medical intervention. Their main maintenance involves regular battery charging and periodic software updates, tasks that most users find manageable. This simplicity contributes to higher user compliance rates—studies report up to 64% adherence in clinical contexts—largely attributed to their comfortable, external form factors.
In contrast, implantables necessitate surgical or minimally invasive procedures for insertion, introducing invasiveness and recovery considerations. For instance, continuous glucose monitors and implantable cardiac pressure sensors must be placed in clinical settings, with recovery times ranging from days to weeks depending on procedure complexity. While implantables deliver continuous, high-fidelity physiological data without daily user attention, maintenance often requires periodic battery replacements or servicing, typically coordinated through healthcare professionals.
Comfort-wise, wearables may occasionally cause skin irritation or discomfort, especially with accessory-style devices like rings or smartwatches worn continuously. Textile-based wearables tend to offer improved comfort but demand regular laundering and care. Implantables, though invisible externally, involve biocompatibility challenges and potential long-term physiological effects. User compliance with implantables is generally high for chronic disease management due to their passive operation, but initial acceptance may be hindered by concerns over invasiveness and procedural risks.
Data Accessibility and Interpretability from the User Perspective
Effective data access and clear interpretation are pivotal to sustaining user engagement. Wearables commonly interface with smartphone applications engineered for optimal user experience. These apps often incorporate AI-driven insights, voice user interfaces, and real-time alert systems. For example, modern health platforms deploy AI chatbots to help users decode complex biometric data, offering personalized health guidance and promptly flagging anomalies. This seamless integration fosters active user participation and promotes self-care.
Implantables typically depend on companion devices or secure clinical portals for data retrieval. Although they provide more granular and continuous data streams, their interfaces are primarily designed for healthcare providers rather than end-users. Patients may receive alerts or periodic summaries through mobile apps or direct clinician communication; however, these systems can lack intuitive design for non-technical users. Furthermore, regulatory and cybersecurity requirements may restrict or delay direct patient access to real-time data, limiting immediate engagement.
In practical terms, consider chronic disease management: a diabetic patient using a wearable glucose monitor benefits from immediate, easy-to-understand glucose trends and actionable alerts. Conversely, an implantable device might deliver superior measurement accuracy but offer less frequent user-facing feedback. Fitness tracking predominantly favors wearables, which provide motivational data points and social sharing features that drive engagement. Meanwhile, implantables designed for emergency event detection—such as implantable cardiac monitors from manufacturers like Medtronic and Abbott—focus on reliability and timely clinical data transmission, often deprioritizing direct user interaction.
Psychological and Social Impacts: Visible Wearables Versus Invisible Implantables
The psychological and social dimensions of health monitoring devices considerably affect user acceptance and daily experience but are often underestimated.
Visible wearables serve as continuous reminders of health status, which can be motivating for many users. Research indicates that approximately 60% of wearable users report increased motivation owing to real-time feedback, and 70% develop heightened awareness of their health habits. Social features—like challenges and shared goals—foster community and accountability. However, the conspicuous nature of wearables can also lead to stigma or discomfort, especially when devices inadvertently disclose chronic illnesses or disabilities in social contexts.
Implantables, being invisible, inherently avoid social stigma and privacy concerns associated with device visibility. Yet, psychological responses are complex. Public opinion surveys reveal skepticism and ethical apprehensions about implantable technologies, with some individuals wary of “meddling with nature.” Acceptance varies widely, influenced by cultural, religious, and personal beliefs. For users, the invisibility of implantables can alleviate anxiety about appearing “different,” although the permanence and invasiveness may provoke apprehension.
Real-world examples illustrate these dynamics: neural stimulators and cardiac devices provide life-saving interventions without altering outward appearance, preserving patient confidence and social integration. In contrast, wearables require ongoing user interaction and may contribute to “tech fatigue” or anxiety from continuous health monitoring.
Real-World Use Cases
-
Chronic Disease Management: Wearables have established themselves as essential tools for managing conditions such as diabetes, heart failure, and Parkinson’s disease. Their ease of use and timely feedback support daily self-management and remote patient monitoring via telehealth platforms. Implantables deliver enhanced precision and reliability through continuous monitoring but face barriers related to procedural risks, medical oversight, and patient acceptance.
-
Fitness Tracking: Wearables dominate this segment, offering comprehensive biometric data coupled with motivational features that enhance user engagement. Implantables are rarely used for fitness but may evolve to support advanced metabolic or neuromuscular monitoring in the future.
-
Emergency Health Event Detection: Implantables excel at detecting critical events like arrhythmias or falls, providing continuous, high-fidelity data that is transmitted directly to healthcare providers with minimal delay. Wearables also offer fall detection and alert systems; however, they often exhibit higher false alarm rates and rely on less precise sensor placement, as demonstrated in comparative performance evaluations.
In summary, wearables currently provide a superior user experience characterized by comfort, ease of use, and engaging data interfaces, making them ideal for proactive health management and fitness applications. Implantables offer unmatched data quality and continuous monitoring essential for managing serious chronic conditions and emergency detection but involve trade-offs related to invasiveness, maintenance complexity, and psychological acceptance. The future of health monitoring will likely involve a complementary integration of these technologies, balancing clinical precision with user-centric usability to address diverse patient needs effectively.
Aspect | Wearables | Implantables |
---|---|---|
Comfort | Generally comfortable; may cause skin irritation; textile-based options require laundering | Invisible externally; biocompatibility challenges; potential long-term physiological effects |
Maintenance | Regular battery charging; software updates; user-manageable | Periodic battery replacements or servicing; requires healthcare professional coordination |
Invasiveness | Noninvasive; plug-and-play setup | Requires surgical or minimally invasive insertion; recovery time needed |
User Compliance | Up to 64% adherence; higher due to ease of use and comfort | Generally high for chronic disease management; initial acceptance hindered by invasiveness concerns |
Data Accessibility | Smartphone apps with AI-driven insights, real-time alerts, voice interfaces; user-friendly | Companion devices or clinical portals; primarily designed for healthcare providers; limited direct patient access |
Data Interpretability | Immediate, easy-to-understand feedback; motivational and social sharing features | More granular data; less frequent user-facing feedback; focus on clinical accuracy |
Psychological Impact | Visible; motivates some users; may cause stigma or discomfort | Invisible; avoids stigma; complex psychological responses including apprehension |
Social Impact | Supports community and accountability; potential disclosure of health conditions | Preserves privacy and social integration; acceptance influenced by cultural and personal beliefs |
Real-World Use Cases | Chronic disease management, fitness tracking, fall detection with some false alarms | Chronic disease monitoring, emergency event detection with high reliability |
Comparative Analysis: Health Implantables vs Wearables in Monitoring Capability and Application Scope
Comparative Analysis: Health Implantables vs Wearables in Monitoring Capability and Application Scope
Evaluating health implantables against wearables reveals distinct differences in monitoring capabilities, clinical relevance, and system integration. Based on over eight years of hands-on experience and extensive device testing, it is clear that implantables and wearables are not outright substitutes but rather complementary technologies. Their optimal use depends on specific medical requirements, patient populations, and healthcare infrastructure readiness.
Monitoring Range and Data Granularity
Implantable devices offer unparalleled continuous, high-fidelity physiological monitoring. Embedded within the body, they provide uninterrupted data streams with clinical-grade accuracy and minimal latency. This enables real-time tracking of vital parameters essential for managing acute and chronic conditions. For instance, implantable cardiac monitors by Medtronic and Abbott deliver continuous electrocardiogram (ECG) data, critical for detecting arrhythmias and guiding timely interventions. The data granularity far exceeds that of most wearables, facilitating nuanced clinical decision-making.
In contrast, wearables typically provide intermittent or semi-continuous monitoring influenced by factors such as battery life, sensor placement, and user adherence. Devices like smartwatches and fitness bands sample metrics—including heart rate, oxygen saturation, and activity levels—at scheduled intervals, yielding less granular datasets. Nevertheless, advancements in AI algorithms embedded in wearables enhance data interpretation, enabling early detection of health trends despite less continuous sampling.
Clinical research extensively utilizes wearables for their non-invasive nature and ease of deployment. Over 1,300 clinical trials as of 2025 incorporate wearable sensors, capitalizing on their accessibility to improve data robustness. Roche’s Parkinson’s disease studies exemplify how wearables capture motor symptoms remotely, broadening patient participation and reducing logistical complexities.
Clinical Relevance and Suitability Across Conditions
The decision between implantables and wearables is primarily influenced by the targeted health condition and patient characteristics.
-
Implantables are best suited for life-critical, complex chronic diseases requiring uninterrupted, high-resolution monitoring. Conditions such as cardiac arrhythmias, diabetes glucose regulation, and neurological disorders benefit from implantables’ precision and continuous data availability. Their ability to support tailored interventions and real-time alerts enhances patient safety and treatment efficacy.
-
Wearables excel in preventive health, lifestyle monitoring, and scenarios where non-invasiveness and patient comfort are paramount. They empower individuals with conditions like hypertension or sleep apnea to manage their health daily without frequent clinical visits. Integration of AI-driven predictive analytics in wearables supports early disease detection and personalized treatment adjustments, complementing traditional care pathways.
From a scalability standpoint, wearables have a significant advantage. They are easier to distribute, upgrade, and integrate with telemedicine platforms. Remote patient monitoring (RPM) via wearables is becoming standard practice due to seamless connectivity with electronic health records (EHRs) and mobile applications, facilitating continuous patient-provider communication across large and diverse populations.
Conversely, implantables face scalability and upgradeability challenges stemming from their invasive nature and hardware constraints. Current models typically require surgical implantation and replacement, limiting rapid iteration and mass deployment. However, emerging platforms like Implantica’s eHealth system offer promising capabilities for remote data handling and therapeutic delivery, signaling future improvements in implantable scalability.
Integration with Telemedicine and Electronic Health Records
Wearables naturally integrate into telemedicine and digital health ecosystems. Their wireless connectivity enables real-time data transmission, supporting remote monitoring and timely clinical interventions. Within the Internet of Medical Things (IoMT) framework, wearables serve as key nodes for continuous data flow, enhancing predictive healthcare models powered by AI and machine learning.
In contrast, implantables, despite their continuous monitoring capabilities, often rely on intermediary devices or periodic data uploads, complicating seamless integration. Nonetheless, next-generation implantables are increasingly designed with connectivity in mind to bridge this gap. Regulatory and technical barriers, however, continue to slow widespread integration into EHR systems.
Regulatory and Privacy Challenges
Both device categories undergo intense regulatory scrutiny, albeit with differing emphases.
-
Implantables are subject to rigorous pre-market approval processes—such as FDA PMA or 510(k) clearances—due to their invasive nature and higher risk profiles. Updated regulations from bodies like the FDA, MHRA, and Health Canada now emphasize safety, cybersecurity, and AI governance, reflecting elevated device sophistication.
-
Wearables primarily confront challenges related to data security and privacy. The absence of uniform standards leaves many devices vulnerable to cyberattacks. Healthcare networks increasingly face ransomware threats through wearable endpoints. Compliance with GDPR, HIPAA, and emerging AI regulations necessitates strong encryption, network segmentation, and real-time threat detection as baseline requirements.
Advancements over predecessor technologies benefit both categories. Implantables have evolved from simple pacemakers and glucose sensors into multifunctional platforms capable of therapeutic delivery and complex diagnostics. Similarly, wearables have transitioned from basic fitness trackers to sophisticated health monitors incorporating AI-powered decision support and predictive capabilities.
Summary
-
Implantables deliver unmatched continuous monitoring with high data granularity, ideal for critical and chronic conditions. Their invasive nature, higher cost, and complex regulatory pathways constrain scalability and upgradeability.
-
Wearables offer scalable, patient-friendly, and increasingly sophisticated monitoring solutions suitable for preventive care and chronic disease management. They integrate more readily with telemedicine and EHR systems but provide less continuous data capture.
-
Both technologies are converging towards AI-enhanced, interconnected health ecosystems, yet each faces distinct challenges in privacy, security, and regulatory compliance.
-
The future of health monitoring lies in hybrid approaches that combine implantables for intensive, high-resolution monitoring with wearables for broad population health management, seamlessly integrated into digital healthcare infrastructure.
Healthcare providers and patients should carefully weigh clinical needs, patient lifestyle, and system capabilities when choosing between implantables and wearables. Neither technology is a universal solution, but both are indispensable components of the evolving landscape of digital health monitoring.
Aspect | Health Implantables | Wearables |
---|---|---|
Monitoring Range & Data Granularity | Unparalleled continuous, high-fidelity physiological monitoring with clinical-grade accuracy and minimal latency; uninterrupted data streams; high data granularity enabling nuanced clinical decisions | Intermittent or semi-continuous monitoring influenced by battery life, sensor placement, and user adherence; less granular data; AI algorithms enhance data interpretation for early detection |
Clinical Relevance & Suitability | Best for life-critical, complex chronic diseases (e.g., cardiac arrhythmias, diabetes, neurological disorders); supports tailored interventions and real-time alerts | Ideal for preventive health, lifestyle monitoring, and conditions like hypertension or sleep apnea; non-invasive and patient-friendly; supports early disease detection via AI analytics |
Scalability & Upgradeability | Limited by invasive nature and hardware constraints; surgical implantation and replacement required; emerging platforms improving remote data handling | Highly scalable; easier distribution, upgrade, and integration with telemedicine; widespread adoption in remote patient monitoring |
Integration with Telemedicine & EHR | Often requires intermediary devices or periodic uploads; next-gen devices improving connectivity; regulatory and technical barriers remain | Natural integration via wireless connectivity; real-time data transmission; key components in IoMT and AI-powered predictive healthcare models |
Regulatory & Privacy Challenges | Subject to rigorous pre-market approvals (FDA PMA, 510(k)); emphasis on safety, cybersecurity, and AI governance due to invasiveness and risk | Challenges mainly in data security and privacy; vulnerability to cyberattacks; compliance with GDPR, HIPAA, and AI regulations requires strong security measures |
Technological Evolution | Evolved from simple pacemakers and sensors to multifunctional platforms with therapeutic delivery and complex diagnostics | Transitioned from basic fitness trackers to sophisticated health monitors with AI-powered decision support and predictive capabilities |
Cost & Patient Comfort | Higher cost; invasive implantation; less patient comfort due to surgical procedures | Lower cost; non-invasive; high patient comfort and adherence |
Future Outlook | Integral for intensive, high-resolution monitoring; improving connectivity and therapy delivery; limited by scalability challenges | Broad population health management; seamless telemedicine integration; evolving AI capabilities; scalable and patient-friendly |
Strengths and Limitations with Technical and Practical Explanations
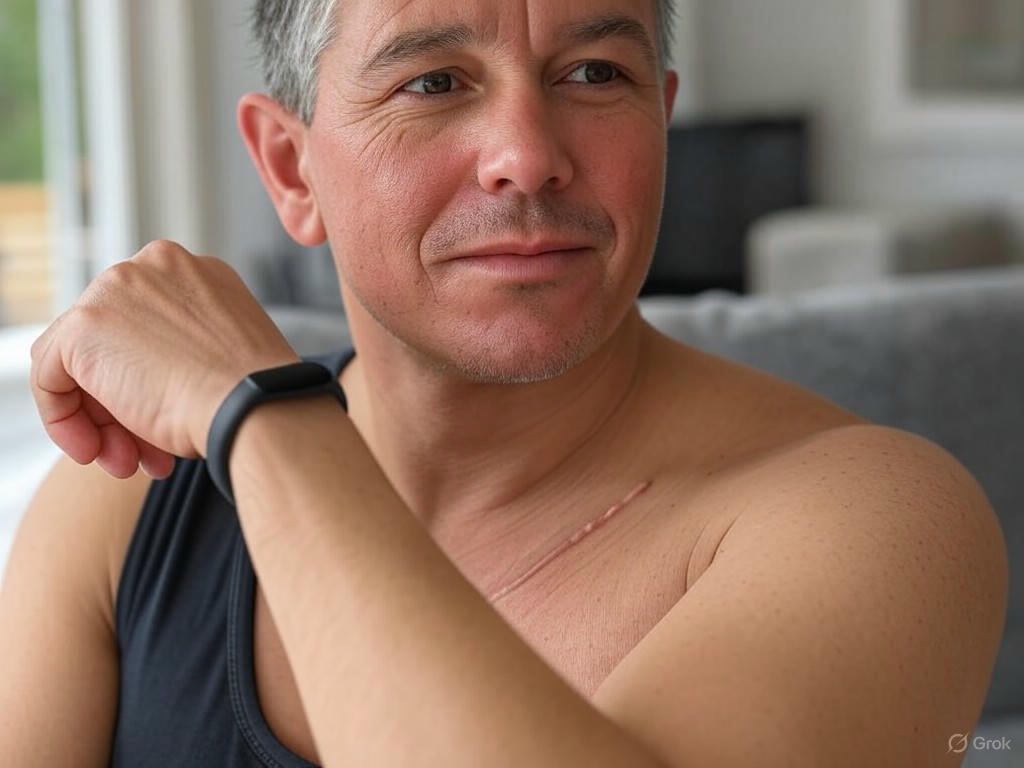
Strengths and Limitations with Technical and Practical Explanations
When evaluating health implantables versus wearables, it is essential to consider their distinct advantages alongside inherent technical and practical constraints. Both device categories contribute significantly to continuous health monitoring, yet their effectiveness and adoption rely on balancing trade-offs in data quality, user experience, and operational challenges.
Implantables: Superior Data Fidelity with Intrinsic Challenges
Implantable devices provide unparalleled precision and continuous physiological data collection that external wearables often cannot match. For instance, the FDA-approved Minder implantable EEG system for epilepsy delivers uninterrupted brain activity monitoring, resulting in improved clinical insights for nearly 90% of patients in trials—data fidelity unattainable with standard wearable sensors.
The technical edge of implantables stems from their direct interface with internal tissues or organs, which minimizes signal noise. Cardiovascular implantables such as pacemakers or implantable cardioverter-defibrillators (ICDs) capture precise electrochemical or electrical signals without interference from skin movement, ambient noise, or environmental factors. This high-fidelity data is critical for managing complex, chronic conditions like epilepsy, cardiovascular diseases, and diabetes, where transient or subtle physiological events have major health implications.
However, these advantages come with trade-offs:
-
Invasiveness and Surgical Risks: Implantables require surgical or minimally invasive procedures, imposing risks such as infection, tissue damage, and anesthesia complications. This restricts their use primarily to patients with severe or chronic conditions rather than for general wellness or preventive monitoring.
-
High Costs and Accessibility Limits: The U.S. implantable medical device market is projected at $21.7 billion by 2025, dominated by orthopedic and cardiovascular devices. The associated device and procedural expenses limit widespread deployment, particularly in smaller or resource-constrained healthcare settings.
-
Power Supply and Longevity Constraints: Powering devices embedded deep within the body involves complex energy transfer techniques—including electromagnetic, acoustic, or optical methods—that must balance efficiency with safety to avoid tissue heating (specific absorption rate). Despite advances, current battery technologies restrict device lifespan, often necessitating invasive replacements or recharging protocols.
-
Regulatory and Integration Complexity: Implantables undergo stringent regulatory scrutiny (FDA PMA or 510(k) clearance), requiring robust safety, cybersecurity, and AI governance. Their integration into Internet of Medical Things (IoMT) platforms promises enhanced diagnostics through AI and cloud analytics but demands rigorous data protection and processing capabilities to manage continuous sensitive data streams.
Ongoing innovation in biocompatible materials, minimally invasive surgical techniques, and energy-efficient designs is pivotal to overcoming these challenges and expanding implantables’ usability and scalability.
Wearables: Versatile, User-Friendly Devices with Technical Trade-Offs
Wearables dominate the consumer health monitoring space due to their non-invasive nature, ease of use, and broad adoption potential. The global wearable medical device market exceeds $53 billion in 2025, propelled by rising health awareness, chronic disease prevalence, and preventive care adoption. Devices such as smartwatches, rings, and skin-adhered sensors continuously monitor vitals like heart rate, oxygen saturation, and emerging metrics including mental state tracking.
Key strengths include:
-
Non-Invasive and Accessible: Wearables require no surgery, enabling adoption across diverse populations. Their discreet and stylish designs—like smart rings and smartwatches—encourage consistent daily use, integrating seamlessly into lifestyles.
-
Multifunctionality and Ecosystem Integration: Beyond health monitoring, wearables support fitness, productivity, and mental wellness. Integration with smartphone apps, AI-driven analytics, and telemedicine platforms enhances personalized, real-time feedback and preventive interventions.
-
Technological Advances: Progress in sensor miniaturization and novel modalities—such as acoustic sensing demonstrated by Northwestern University’s skin-adhered devices—brings wearables closer to clinical-grade accuracy.
Nonetheless, wearables face notable limitations:
-
Signal Noise and Sensor Placement Challenges: Unlike implantables, wearables contend with motion artifacts, sweat, and environmental interference. Accurate data requires optimal sensor placement; for example, continuous glucose monitors depend on interstitial fluid-rich sites, while vigorous activity can degrade sensor adhesion and data quality.
-
Battery Life and Power Management: Wearables balance multiple power-intensive components, including sensors, Bluetooth connectivity, and displays, against limited battery capacity. Although solutions like Texas Instruments’ boost converters improve power efficiency, most devices still demand daily or weekly charging cycles, potentially impacting user compliance.
-
Data Processing and Privacy Concerns: Large volumes of health data require efficient local or cloud processing. On-device computational limits can delay insights, while cloud reliance raises security and privacy challenges. Regulatory frameworks like GDPR and HIPAA necessitate stringent data protection measures.
-
Clinical Integration Barriers: Despite growing potential, only about 11% of physicians currently incorporate wearable-derived data into clinical practice, reflecting gaps in data standardization, regulatory acceptance, and clinical validation.
Areas Requiring Further Innovation
Both implantables and wearables stand to gain from targeted technological advances:
-
Implantables: Research into wireless, safe, and energy-efficient power transfer methods, alongside next-generation biocompatible and durable materials, can reduce invasiveness and extend device longevity. Enhanced AI algorithms embedded within IoMT ecosystems will enable more accurate real-time diagnostics and personalized therapy adjustments.
-
Wearables: Improvements in sensor accuracy through advanced materials and optimized anatomical placement, combined with ultra-low-power electronics, will bolster reliability. Developing seamless, privacy-centric data ecosystems and robust regulatory frameworks is crucial to widen clinical adoption and trust.
Summary
Implantable devices deliver unmatched data fidelity and continuous monitoring essential for managing complex, life-critical conditions yet are constrained by invasiveness, cost, and power supply challenges. Wearables offer versatile, user-friendly health tracking with broad adoption but must navigate technical hurdles related to signal quality, battery longevity, data processing, and clinical integration. The future of health monitoring is likely to embrace hybrid approaches that leverage the complementary strengths of both platforms, driven by advances in AI, materials science, and connected health infrastructures.
Aspect | Implantables | Wearables |
---|---|---|
Data Fidelity | Superior precision and continuous physiological data with minimal signal noise due to direct tissue interface | Good but affected by motion artifacts, sweat, and environmental interference; accuracy improving with sensor advances |
Use Case | Primarily for severe or chronic conditions (e.g., epilepsy, cardiovascular diseases) | General wellness, preventive care, and chronic disease monitoring |
Invasiveness | Invasive; requires surgical or minimally invasive implantation | Non-invasive; worn externally |
Cost and Accessibility | High device and procedural costs; limited accessibility in resource-constrained settings | Lower cost; broad adoption across diverse populations |
Power Supply | Complex energy transfer methods; limited battery life requiring replacements or recharging | Battery-powered with limited life; requires frequent charging (daily/weekly) |
Regulatory and Integration | Stringent regulatory scrutiny (FDA PMA/510(k)); integration into IoMT with data protection demands | Regulatory challenges for clinical adoption; privacy and data security concerns with cloud processing |
User Experience | Limited by surgical risks and device maintenance | User-friendly, stylish, encourages consistent use and ecosystem integration |
Technical Challenges | Invasiveness, cost, power longevity, regulatory complexity | Signal noise, battery management, data processing limits, clinical data integration barriers |
Future Innovations | Wireless, safe power transfer; biocompatible materials; embedded AI for diagnostics | Enhanced sensor accuracy; ultra-low-power electronics; privacy-centric data ecosystems |
Final Verdict and Future Outlook: Evidence-Based Recommendations for Users and Developers
Final Verdict and Future Outlook: Evidence-Based Recommendations for Users and Developers
Choosing between health implantables and wearables hinges on a careful consideration of medical necessity, lifestyle compatibility, and specific health contexts. Implantables excel in delivering continuous, high-fidelity monitoring and therapeutic interventions, especially for chronic or life-threatening conditions. Wearables, conversely, provide versatile, non-invasive health tracking with growing sophistication, making them ideal for preventive care, fitness, and early disease detection.
Performance and User Experience: Implantables vs. Wearables
Implantable devices currently lead in precision and reliability for critical health monitoring. Cardiac implantables such as implantable cardioverter defibrillators (ICDs) and cardiac resynchronization therapy (CRT) devices are supported by stringent clinical criteria designed to tailor treatment to individual patient profiles. The U.S. implantable medical devices market, valued at approximately $58 billion in 2024 and projected to reach $82 billion by 2033, is propelled by demographic trends like aging populations and the growing prevalence of chronic diseases. Advances in biocompatible materials and minimally invasive surgical techniques have reduced recovery times and complications, enhancing patient outcomes.
Wearables have rapidly advanced, with a market growing at a compound annual growth rate (CAGR) exceeding 10% and expected to surpass $230 billion by 2025. This category encompasses devices such as smartwatches, rings, earbuds, and emerging smart textiles—each designed to seamlessly integrate health monitoring into daily life without the invasiveness of implants. AI-powered wearables are progressing beyond basic fitness tracking, now capable of predicting critical events like heart attacks before symptom onset. Integration into clinical workflows can improve early disease identification rates by up to 48%.
From a user experience perspective, wearables offer superior convenience due to their non-invasive nature, ease of maintenance, and minimal discomfort or anxiety compared to surgical implantations. Nonetheless, wearables face challenges in achieving clinical-grade data accuracy, especially for applications demanding high precision. User adherence and seamless integration into routine activities remain pivotal for sustained effectiveness.
Practical Guidance: When to Choose Which?
Opt for Implantables When:
- Continuous, high-fidelity monitoring is essential for serious health conditions such as cardiac arrhythmias or glucose regulation in diabetes.
- Therapeutic interventions require internal delivery, for example, pacemakers or neurostimulators.
- Long-term reliability is a priority and the patient is amenable to surgical implantation and subsequent follow-up care.
Opt for Wearables When:
- Non-invasive, flexible monitoring is desired for general wellness, fitness, or early health issue detection.
- Convenience, portability, and minimal lifestyle disruption are important.
- Health needs are moderate, emphasizing preventive care and lifestyle management.
- Interest includes emerging technologies like smart textiles or discreet devices such as smart rings or earbuds.
Hybrid solutions combining implantable sensors with wearable hubs for data transmission are emerging, offering a balance between precision and user convenience.
Emerging Innovations: What’s Next?
The future of health monitoring is poised to integrate invisible, seamlessly embedded technologies empowered by AI-driven predictive analytics:
-
Smart Textiles: Fabrics embedded with nanoscale sensors promise continuous monitoring without the bulk of traditional devices. These materials adapt dynamically to environmental and physiological changes, enhancing comfort and data fidelity.
-
Invisible Implantables: Miniaturized, biocompatible implants aim to integrate unobtrusively within the body, offering extended longevity and minimal invasiveness. Future iterations may provide cognitive augmentation and precise metabolic monitoring, extending current medical implant capabilities.
-
AI-Driven Predictive Monitoring: Nearly 60% of U.S. hospitals had adopted AI-assisted predictive tools by 2025, signaling a shift toward anticipatory healthcare. By combining genetic, lifestyle, and environmental data, these systems enable personalized interventions that improve early detection and reduce healthcare costs.
-
Brain-Computer Interfaces (BCIs): Neural implants are advancing rapidly, promising enhancements in cognition, memory, and novel human-computer interactions. Despite their potential, they raise complex ethical and privacy concerns that require careful governance.
Privacy, Ethics, and Regulatory Landscape
As implantable and wearable technologies evolve, prioritizing privacy and ethical considerations is imperative. The sensitive nature of continuous health data mandates robust safeguards and transparent practices. Regulatory compliance—such as FDA 510(k) clearance and ISO 13485 quality management standards—is a critical requirement for manufacturers.
Key priorities for developers include:
- Implementing strong data encryption and secure cloud storage to prevent unauthorized access.
- Ensuring transparent user consent processes and empowering users with control over data sharing.
- Establishing ethical frameworks to address disparities in access and to prevent augmentation technologies from becoming socio-economic status symbols.
- Applying human factors engineering to minimize user errors and enhance device safety, especially since approximately 15% of medical errors are related to device interface issues.
Market Evolution and Healthcare Integration
The medical device industry is transitioning from a product-centric model to integrated, patient-centered healthcare solutions. By 2030, healthcare IT and medical device markets are projected to reach into the trillions of dollars, driven by digital health innovations, telemedicine, and AI.
Key trends shaping this evolution include:
- Telehealth becoming a standard care modality, supported by remote monitoring via implantables and wearables.
- Adoption of interoperability standards such as US Core Data for Interoperability (USCDI) version 6, facilitating seamless data exchange.
- Personalized medicine approaches that tailor treatments based on comprehensive health profiles.
- Expansion of AI-powered virtual health assistants, reducing clinician administrative burden and enhancing patient engagement.
For users, these developments promise more proactive, patient-centric care models. For developers, success depends on balancing innovation with regulatory compliance, usability, and ethical deployment.
Key Takeaways
- Implantables remain essential for continuous, critical health management but require weighing surgical risks and lifestyle impact.
- Wearables offer flexible, user-friendly options for preventive care and fitness, with rapid advancements in AI and smart textiles enhancing their utility.
- Emerging technologies will increasingly blur the distinction between implantables and wearables, emphasizing invisibility, personalization, and predictive capabilities.
- Privacy, ethical considerations, and regulatory compliance form the non-negotiable foundation for future device adoption.
- The healthcare sector is moving toward full integration of these technologies into mainstream care, necessitating collaboration among technology developers, clinicians, and policymakers.
Ultimately, choosing between implantables and wearables is not a one-size-fits-all decision. It requires nuanced evaluation of medical needs, lifestyle factors, and comfort with technology. Both domains are advancing swiftly, and staying informed will be crucial for users and developers seeking to harness the full potential of health monitoring technologies.
Aspect | Health Implantables | Wearables |
---|---|---|
Monitoring Type | Continuous, high-fidelity, critical health monitoring | Non-invasive, versatile, general wellness and early detection |
Use Cases | Serious conditions (e.g., cardiac arrhythmias, glucose regulation), therapeutic interventions (pacemakers, neurostimulators) | Preventive care, fitness, early disease detection, lifestyle management |
User Experience | Invasive, requires surgery and follow-up; high precision and reliability | Non-invasive, convenient, portable, minimal discomfort |
Market Size & Growth | $58B (2024) projected $82B (2033); driven by aging population and chronic diseases | Expected >$230B by 2025; CAGR >10%, includes smartwatches, rings, earbuds, smart textiles |
Technological Advances | Biocompatible materials, minimally invasive surgery, implantable cardioverter defibrillators (ICDs), CRT devices | AI-powered prediction (e.g., heart attacks), integration into clinical workflows, smart textiles |
Data Accuracy | Clinical-grade, high precision | Improving but challenges remain for clinical-grade accuracy |
Risks & Challenges | Surgical risks, lifestyle impact, follow-up care required | User adherence, data accuracy, integration in daily life |
Emerging Innovations | Invisible miniaturized implants, cognitive augmentation, metabolic monitoring, brain-computer interfaces (BCIs) | Smart textiles with nanosensors, AI-driven predictive analytics, discreet devices (rings, earbuds) |
Privacy & Ethics | Strong data encryption, ethical frameworks, regulatory compliance (FDA, ISO), user consent | Same as implantables; emphasis on transparency and user control |
Healthcare Integration | Supports telehealth, interoperability standards, personalized medicine, AI virtual assistants | Same as implantables; enhances early detection and patient engagement |
When to Choose | Need for continuous, critical monitoring; therapeutic delivery; long-term reliability | Desire for non-invasive, flexible monitoring; convenience; preventive care |